EV-idence uncovered: kidney-on-chip study links circulating EVs of cardiorenal syndrome to renal pathology
Abstract
The intertwined nature of cardiac and renal failure, where dysfunction in one organ predicts a poor outcome in the other, has long driven the interest in uncovering the exact molecular links between the two. Elucidating the mechanisms driving Cardiorenal Syndrome (CRS) will enable the development of targeted therapies that disrupt this detrimental cycle, potentially improving outcomes for patients. A recent study by Chatterjee et al. (JCI insight 2023) demonstrated the feasibility of utilizing a humanized microfluidic kidney-on-chip model to elucidate the role of circulating extracellular vesicles (EVs) in the development of CRS (type 1 and type 2) in heart failure (HF) patients. The study also identified and validated EV miRNAs that correlated with kidney function by targeting several genes involved in kidney damage pathways, including transforming growth factor- β (TGF-β) signaling. These findings suggest that plasma EVs from CRS patients induce harmful responses in renal cells by regulating key pathways, highlighting their role in both type 1 and type 2 CRS.
Keywords
COMMENTARY
Cardiorenal syndrome (CRS) is a complex condition in which heart and kidney dysfunction are closely linked, increasing the overall morbidity and mortality of these patients. Notably, the presence of both heart failure (HF) with preserved ejection fraction (HFpEF) and chronic kidney disease (CKD) have a poor prognosis due to synergistic effects. Thus, understanding mechanisms associated with CRS is crucial for developing targeted therapies to improve outcomes and reduce healthcare burden. Circulating extracellular vesicles (EVs), small membrane-bound particles carrying a diverse array of molecular cargo, including proteins, lipids, and nucleic acids, play a pivotal role in facilitating systemic EV-mediated communication. Through their molecular payload, EVs can modulate recipient cell behavior, influencing various physiological and pathological processes. Despite their emerging importance in trans-organ communication, the specific role of circulating EVs in CRS remains unknown. In a recent study by Chatterjee et al., a “Kidney on Chip”[1] (Emulate. Inc) device was used to investigate the role of EVs in kidney damage associated with HFpEF, shedding light on EV-based mechanisms of renal injury[2]. This innovative approach explores the kidney-circulatory system interface, revealing how EVs contribute to renal injury in HFpEF [Figure 1].
Figure 1. A graphical representation of Renal Injury via Circulating EVs in CRS-HFpEF: A Kidney-on-Chip Analysis (created with BioRender.com). EVs: Extracellular vesicles; CRS: cardiorenal syndrome; HFpEF: heart failure with preserved ejection fraction.
The pathophysiology of CRS is complex and not fully understood due to several reasons. Multiple mechanisms such as the renin-angiotensin system, oxidative stress, inflammation, and sympathetic nervous system have been identified as associated factors[3-5], but the precise mechanism remains elusive due to the unavailability of reproducible rodent models. Moreover, there are limitations to using nonhuman primate models due to ethical concerns, high costs, and the risk of zoonotic infections hindering research[6]. The development of kidney organoids is obstructed by intricate renal functions and requirements for precise vascularization, drainage integration, and in vivo-like microenvironment recapitulation[3]. To overcome this, Das group used a human kidney-on-chip model to study trans-organ communication in renal injury mediated by HFpEF patient-derived circulating EVs, offering a translatable approach to understand this complex relationship. Chatterjee et al. demonstrated that EVs derived from patients with CRS induce significantly greater renal cell injury compared with EVs from HFpEF patients without CRS[2]. This increased injury was evidenced by elevated levels of injury biomarkers, including interleukin-18 (IL-18), kidney injury molecule-1 (KIM-1), and neutrophil gelatinase-associated lipocalin (NGAL). Furthermore, analysis of kidney-on-chip effluents revealed alterations in cystatin C protein expression, a constitutive biomarker of CKD. Changes in cystatin C levels in effluents may parallel in vivo fluctuations in circulating cystatin C, reflecting renal function alterations. Notably, the study also revealed activation of profibrotic pathways, specifically transforming growth factor-β (TGF-β) pathway, leading to epithelial-to-mesenchymal transition (EMT) and fibrogenesis. This exacerbates kidney injury, highlighting the potential role of EV-mediated communication in CRS pathogenesis.
EV-derived miRNAs may induce transcriptional and functional changes in recipient cells, suggesting a key role in renal injury[3,7,8]. Therefore, establishing a direct link between EV-mediated miRNA signaling and renal injury has the potential to uncover new therapeutic targets. This work identified 78 differentially expressed miRNAs between control and HFpEF patients. Of these, 15 miRNAs were directly associated with circulating creatinine levels, as indicated by the elastic net analysis. These 15 miRNAs targeted 1,143 genes. Pathway analysis revealed enrichment in 35 Kyoto Encyclopedia of Genes and Genomes (KEGG) biological processes, including AMP-activated protein kinase (AMPK) signaling, cell cycle, TGF-β signaling, and
EVs in circulation may originate from many different organs[9], and based on the deconvolution analysis of RNA content of EVs (with respect to tissue atlases of RNA), methods to understand the origin of EVs can be explored[10]. However, given the promiscuous expression of miRNAs, the assessment of EV long RNA transcriptome is a better tool to decipher the tissue origin of EVs in the circulation (mRNAs and lncRNAs have better tissue specificity than miRNAs). The deconvolution analysis from Das group’s study reveals that EVs likely originate from the heart, skeletal muscle, adipose tissue, liver and bone marrow (immune cells), with no significant differences between HFpEF CRS and non-CRS groups, suggesting that the miRNA contents of EVs drive disease pathology, rather than its origin. This indicates that circulating EV miRNA profiling provides a non-invasive approach for monitoring CRS disease progression and therapeutic response during HFpEF, addressing the limitations of traditional biomarkers in this heterogeneous disease[11].
STRENGTHS, LIMITATIONS AND FUTURE DIRECTIONS
The study by Chatterjee et al. is substantially strengthened by leveraging human-derived EVs, cells, and particularly the innovative kidney-on-chip model[2]. This approach enables a more accurate representation of the complex interplay between organs and EV-mediated communication in CRS. By utilizing this cutting-edge model, the authors aimed to bridge the gap between traditional in vitro studies and in vivo complexity, providing valuable insights into the molecular mechanisms underlying CRS. Moreover, currently, no established benchmark or study defines the optimal or excessive levels of EV uptake in cells, particularly in vivo. Nevertheless, Chatterjee et al.’s study[2] provides valuable insights by calculating EV exposure per cell. Notably, their calculations reveal EV exposure levels (6,000 EVs/cell over 72 h) that fall within ranges previously reported to elicit physiological effects[12]. Despite its strengths, the study is not without limitations. One major challenge is the inherent complexity of EV biology. EVs are heterogeneous, encompassing a range of particles, including exosomes, microvesicles, and apoptotic bodies, each with distinct biogenesis pathways and cargo. The use of complementary isolation techniques, while enhancing EV specificity, does not entirely preclude the co-isolation of non-EV entities. Therefore, more refined purification approaches are essential to ensure that observed biological effects are exclusively attributable to EVs, rather than to other plasma components that may be present in the isolated fraction. The study’s reliance on in vitro models is another limitation, as they cannot fully capture the intricate physiological processes occurring in living organisms. Although the Kidney-on-chip model is sophisticated, it lacks the cellular diversity and dynamic interactions present in vivo. Furthermore, the study does not investigate EV biodistribution in living systems, which is a critical factor in evaluating their therapeutic potential. Consequently, future studies should aim to validate these findings in animal models and, ultimately, in human clinical trials. The study also raises important questions regarding the generalizability of its findings. While the focus on HFpEF provides valuable insights, it is unclear whether the same mechanisms apply to other forms of HF, such as HF with reduced ejection fraction (HFrEF). Further investigation is needed to determine if the identified miRNA signatures are unique to CRS or represent a more universal response to kidney damage. The functional assays employed in this study are restricted to EV uptake and biomarker expression, without direct measurement of cellular behavior alterations. Future research can address this limitation by incorporating additional assays to evaluate cell migration, proliferation, signaling pathways, and functional process analysis, which will provide a more comprehensive understanding of EV-induced effects. Although Chatterjee et al. demonstrate that circulating EV miRNAs in CRS patients exert chronic harmful effects on renal cells via TGF-β pathway regulation, the study does not differentiate between type 1 and type 2 CRS, implying possible shared mechanisms[2]. Moreover, the clinical relevance of EVs in CRS is still underexplored. While EVs have been identified as potential biomarkers for CRS, their diagnostic and prognostic value in human patients remains unclear due to a lack of large-scale clinical studies. The specificity and sensitivity of EVs as biomarkers need to be thoroughly validated in diverse patient populations, considering factors such as age, gender, and comorbidities. Additionally, the therapeutic potential of EVs, while promising, is hindered by the lack of targeted delivery methods and concerns regarding the safety and scalability of EV-based therapies. Finally, the exact mechanism of how EVs are taken up by the cells and their molecular cargos reach their final destination [e.g., loading onto RNA-induced silencing complex (RISC) complex for miRNAs] is still unclear. Nonetheless, the use of the miRNA mimics and inhibitors to oppose or replicate the effects of the CRS-EV miRNA cargo argues for the functional transfer of these miRNAs and silencing of their target mRNAs.
CONCLUSION
Studying EVs in CRS offers promising opportunities for advancing our understanding and treatment of the disease. The framework established by Das group’s study provides a robust foundation for investigating EV-mediated communication in multiorgan diseases. However, to fully leverage EVs, we must develop standardized methods, conduct rigorous clinical trials, and explore innovative therapies to overcome existing challenges and unlock their potential in CRS research and treatment.
DECLARATIONS
Authors’ contributions
Wrote the manuscript: Ndiaye H
Participated in helping to write the manuscript: Rajput S, Sauld JFK, Mahajan G
Conceived and designed the idea of the manuscript and assisted in preparing the manuscript: Das S, Chatterjee E
Be responsible for overall supervision: Chatterjee E
Availability of data and materials
Not applicable.
Financial support and sponsorship
This study was supported by the American Heart Association (AHA) (SFRN16SFRN31280008) and the National Heart, Lung, and Blood Institute (1R35HL150807-01).
Conflicts of interest
All authors declared that there are no conflicts of interest.
Ethical approval and consent to participate
Not applicable.
Consent for publication
Not applicable.
Copyright
© The Author(s) 2024.
REFERENCES
1. Jang KJ, Mehr AP, Hamilton GA, et al. Human kidney proximal tubule-on-a-chip for drug transport and nephrotoxicity assessment. Integr Biol (Camb) 2013;5:1119-29.
2. Chatterjee E, Rodosthenous RS, Kujala V, et al. Circulating extracellular vesicles in human cardiorenal syndrome promote renal injury in a kidney-on-chip system. JCI Insight 2023;8:e165172.
3. Bongartz LG, Cramer MJ, Doevendans PA, Joles JA, Braam B. The severe cardiorenal syndrome: ‘Guyton revisited’. Eur Heart J 2005;26:11-7.
4. Ronco C, Haapio M, House AA, Anavekar N, Bellomo R. Cardiorenal syndrome. J Am Coll Cardiol 2008;52:1527-39.
6. Becker GJ, Hewitson TD. Animal models of chronic kidney disease: useful but not perfect. Nephrol Dial Transplant 2013;28:2432-8.
7. Sepúlveda F, Mayorga-Lobos C, Guzmán K, Durán-Jara E, Lobos-González L. EV-miRNA-mediated intercellular communication in the breast tumor microenvironment. Int J Mol Sci 2023;24:13085.
8. Valkov N, Das A, Tucker NR, et al. SnRNA sequencing defines signaling by RBC-derived extracellular vesicles in the murine heart. Life Sci Alliance 2021;4:e202101048.
9. Alberro A, Iparraguirre L, Fernandes A, Otaegui D. Extracellular vesicles in blood: sources, effects, and applications. Int J Mol Sci 2021;22:8163.
10. Li Y, He X, Li Q, et al. EV-origin: enumerating the tissue-cellular origin of circulating extracellular vesicles using exLR profile. Comput Struct Biotechnol J 2020;18:2851-9.
11. Carris NW, Mhaskar R, Coughlin E, Bracey E, Tipparaju SM, Halade GV. Novel biomarkers of inflammation in heart failure with preserved ejection fraction: analysis from a large prospective cohort study. BMC Cardiovasc Disord 2022;22:221.
Cite This Article

How to Cite
Ndiaye, H.; Rajput S.; Sauld J. F. K.; Mahajan G.; Das S.; Chatterjee E. EV-idence uncovered: kidney-on-chip study links circulating EVs of cardiorenal syndrome to renal pathology. Extracell. Vesicles. Circ. Nucleic. Acids. 2024, 5, 674-9. http://dx.doi.org/10.20517/evcna.2024.70
Download Citation
Export Citation File:
Type of Import
Tips on Downloading Citation
Citation Manager File Format
Type of Import
Direct Import: When the Direct Import option is selected (the default state), a dialogue box will give you the option to Save or Open the downloaded citation data. Choosing Open will either launch your citation manager or give you a choice of applications with which to use the metadata. The Save option saves the file locally for later use.
Indirect Import: When the Indirect Import option is selected, the metadata is displayed and may be copied and pasted as needed.
About This Article
Copyright
Data & Comments
Data
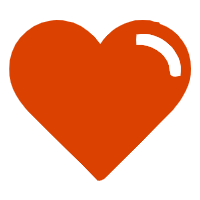
Comments
Comments must be written in English. Spam, offensive content, impersonation, and private information will not be permitted. If any comment is reported and identified as inappropriate content by OAE staff, the comment will be removed without notice. If you have any queries or need any help, please contact us at support@oaepublish.com.