Full-performance coordinated design for polymer-in-salt solid electrolyte
Solid-state lithium batteries (SSLBs) using solid electrolytes have been identified as one of the most promising next-generation energy storage devices due to their improved safety and high energy density. In particular, solid polymer electrolytes (SPEs; excluding gel polymer electrolytes and polymer electrolytes with free solvents) have been widely chosen in SSLBs due to their high lithium metal compatibility, good contact with electrodes, and more favorable manufacturing process[1-3]. Nonetheless, there are still key challenges that SSLBs with SPEs must overcome. These include low ionic conductivity (10-7-10-5 S cm-1) at room temperature and the difficult balance among mechanical strength, ionic conductivity, and high-voltage applications for long-term cycling[4]. Polymer-in-salt solid electrolytes (PISSEs), defined as a small amount of a high-molar-mass polymer mixed with high fraction of lithium salts (generally exceeding 50 wt%), have been proposed to address the ionic conductivity issue[5]. By using PISSEs, the ionic conductivity of typical SPEs can be increased by an order of magnitude (promoted to 10-4 S cm-1), since PISSEs can construct a unique Li+ transport channel through the ion clusters in the system, compared with the Li+ migration in ordinary SPEs that only depends on the segmental movement of the polymer matrix.
However, two key issues of performance regulation continue to hinder the further development of PISSEs. On the one hand, the mechanical property is typically deteriorated with ultrahigh concentration of lithium salt. For example, when the weight ratio of lithium salt is as high as 1.3, the tensile stress of PISSE is only
To address the above two issues, Zhang et al. recently proposed the concept of “local high concentration” in PISSEs[13], which is analogous to that in traditional organic electrolytes and different from the “high concentration” design in traditional PISSEs[14-22] [Figure 1]. The traditional local high concentration liquid electrolyte (LHCE) consists of an inert diluent and original high concentration electrolyte (HCE). The diluent must be easily miscible with the solvating solvent in HCEs, but does not participate in dissociating salts. Therefore, the salt content and electrolyte viscosity per unit volume can be lowered while maintaining the coordination of HCE's original salt and solvent. The new PISSE is based on a polymer blend that is called Li-polymer in F diluter (LPIFD). In LPIFD, PVDF-HFP, an inert fluorinated polymer (called the F diluter; FD), is utilized because of its outstanding mechanical properties and high stability with lithium metal and high-voltage cathodes, while Li-polymer (LP) is PISSE, equivalent to HCE, providing continuous Li+ conduction channels. For the selection of LP, the authors discussed five polymers: Poly(propylene carbonate) (PPC), Poly(ethylene oxide) (PEO), Poly(methyl methacrylate) (PMMA), Poly(vinyl sulfone) (PVSF), and Poly(bis(trifluoroethoxy)phosphazene) (PTFEP). It was revealed that the two fluorinated polymers, PVSF and PTFEP, can exhibit superior miscibility with PVDF-HFP, forming a stable, single-phase structure. Furthermore, PTFEP and PVDF-HFP were selected to construct the PTFEP-LPIFD electrolyte for in-depth investigations.
The results showed that this electrolyte not only inherited excellent lithium-ion conductivity of PISSE, but also showcased significant advancements in two critical indicators: mechanical properties and stability for high-voltage cathodes, demonstrating a high comprehensive performance as compared to traditional PISSEs[14-22]. In detail, PTFEP-LPIFD possessed a high room-temperature ionic conductivity of
The built-in PTFEP-LPIFD in Li||Cu half cells further demonstrated exceptional compatibility with Li anodes. At 0.5 mA cm-2, the Li||Cu cell exhibited a CE of 99.1% after 300 cycles. Additionally, the Li anode showed a dense and uniform surface structure after plating at 2.0 mAh cm-2, which arises from the homogeneous, organic-less and LiF-rich SEI in PTFEP-LPIFD suppressing dendritic Li growth[23].
The full-performance coordinated design for PISSE by Zhang et al. was based on the straightforward blade-coating technique, which is easy to scale and developed as a low-cost, scalable, and controllable manufacturing technique[13]. Nevertheless, there are still certain limitations in this work, which also exist in most other PISSE systems. Firstly, the selection of solvent in this electrolyte still leaves considerable room for improvement. It was demonstrated that there were small residual amounts of 1,2-Dimethoxyethane (DME) solvent that can enhance the ionic conductivity of PISSEs. However, the challenges related to interface contact degradation induced by such highly polar solvents require further refinement[24]. Secondly, the selection of the two polymers for the construction of the LPIFD system is still empirical. In this regard, utilizing computational methods in materials science such as density functional theory (DFT) and molecular dynamics (MD) can help evaluate the microstructure and some key physical/chemical properties of PISSEs, facilitating optimal selection of polymers, salts, and salt concentrations[25]. In addition, high-throughput computing (HTC), which has recently received significant attention, can rapidly screen a large number of material combinations, efficiently providing theoretical guidance and potential solutions[26]. These avant-garde computational tools are poised to shorten cycles of scientific understanding and technological innovation, aiding in the realization of more efficient, stable and durable PISSEs. Thirdly, high-energy-density SSLBs have not been demonstrated at the device level. To enable the practical application of PISSE in SSLBs in the future, key techniques to reduce the thickness of PISSE while maintaining the electrochemical attributes are highly desired. With further pairing with high mass-loading cathodes and addressing the interfacial issues of solid-solid contact, PISSE-based high energy SSLBs can be anticipated.
DECLARATIONS
Authors’ contributions
Proposed the topic: Ma, Q.
Performed literature survey: Shen, S.; Lu, J.
Prepared the manuscript: Ma, Q.; Shen, S.; Ai, L.
Collectively discussed and revised the manuscript: Ma, Q.; Shen, S.; Lu, J.; Ai, L.; Hou, Y.
Review, conceptualization, and supervision: Liu, J.; Li, Y.
Availability of data and materials
Not applicable.
Financial support and sponsorship
This work was supported by grants from the National Natural Science Foundation of China (Nos. 52072136, 52172229, 52272201, 52472247, 51972257), Yanchang Petroleum-WHUT Joint Program (No. yc-whlg-2022ky-05) and Fundamental Research Funds for the Central Universities (Nos. 104972024RSCrc0006, 2023IVA106) for financial support.
Conflicts of interest
Liu, J. is the Guest Editor of the Special Issue “Solid-State Lithium Batteries: Material Innovations and Future Perspectives”, while the other authors have declared that they have no conflicts of interest.
Ethical approval and consent to participate
Not applicable.
Consent for publication
Not applicable.
Copyright
© The Author(s) 2025.
REFERENCES
1. Liu, M.; Cheng, Z.; Ganapathy, S.; et al. Tandem interface and bulk Li-ion transport in a hybrid solid electrolyte with microsized active filler. ACS. Energy. Lett. 2019, 4, 2336-42.
2. Xu, K. Electrolytes and interphases in Li-ion batteries and beyond. Chem. Rev. 2014, 114, 11503-618.
3. Yao, Y.; Wei, Z.; Wang, H.; et al. Toward high energy density all solid-state sodium batteries with excellent flexibility. Adv. Energy. Mater. 2020, 10, 1903698.
4. Cao, X.; Ren, X.; Zou, L.; et al. Monolithic solid-electrolyte interphases formed in fluorinated orthoformate-based electrolytes minimize Li depletion and pulverization. Nat. Energy. 2019, 4, 796-805.
5. Angell, C. A.; Liu, C.; Sanchez, E. Rubbery solid electrolytes with dominant cationic transport and high ambient conductivity. Nature 1993, 362, 137-9.
6. Liu, W.; Yi, C.; Li, L.; et al. Designing polymer-in-salt electrolyte and fully infiltrated 3D electrode for integrated solid-state lithium batteries. Angew. Chem. Int. Ed. 2021, 60, 12931-40.
7. Wang, Y.; Li, T.; Yang, X.; et al. 2D solid-electrolyte interphase built by high-concentration polymer electrolyte for highly reversible silicon anodes. Adv. Energy. Mater. 2024, 14, 2303189.
8. Qiu, J.; Liu, X.; Chen, R.; et al. Enabling stable cycling of 4.2 V high-voltage all-solid-state batteries with PEO-based solid electrolyte. Adv. Funct. Mater. 2020, 30, 1909392.
9. Shi, C.; Yu, M. Flexible solid-state lithium-sulfur batteries based on structural designs. Energy. Storage. Mater. 2023, 57, 429-59.
10. Zhou, Q.; Fu, C.; Li, R.; et al. Poly (vinyl ethylene carbonate)-based dual-salt gel polymer electrolyte enabling high voltage lithium metal batteries. Chem. Eng. J. 2022, 437, 135419.
11. Zhu, M.; Wu, J.; Liu, B.; et al. Multifunctional polymer electrolyte improving stability of electrode-electrolyte interface in lithium metal battery under high voltage. J. Membr. Sci. 2019, 588, 117194.
12. Wang, J.; Yamada, Y.; Sodeyama, K.; Chiang, C. H.; Tateyama, Y.; Yamada, A. Superconcentrated electrolytes for a high-voltage lithium-ion battery. Nat. Commun. 2016, 7, 12032.
13. Zhang, W.; Koverga, V.; Liu, S.; et al. Single-phase local-high-concentration solid polymer electrolytes for lithium-metal batteries. Nat. Energy. 2024, 9, 386-400.
14. Chen, A.; Zeng, Q.; Wen, W.; et al. A highly salt-soluble ketone-based all-solid-state polymer electrolyte with superior performances for lithium-ion batteries. ACS. Appl. Mater. Interfaces. 2023, 15, 17791-800.
15. Yang, J.; Li, R.; Zhang, P.; et al. Crosslinked polymer-in-salt solid electrolyte with multiple ion transport paths for solid-state lithium metal batteries. Energy. Storage. Mater. 2024, 64, 103088.
16. You, D.; Farooq, M. A.; Lai, Z.; Wei, W.; Xiong, H. In-built curable high-salt-concentrated elastomeric polymer electrolytes for integrated all-solid-state lithium batteries and ion conduction. Macromolecules 2024, 57, 4471-83.
17. Ma, Q.; Liu, D.; Wang, B.; Liu, W.; Xiong, G.; Liu, J. Cellulose acetate-promoted polymer-in-salt electrolytes for solid-state lithium batteries. J. Solid. State. Electrochem. 2023, 27, 1411-21.
18. Le Nguyen, M.; Nguyen, V. C.; Lee, Y. L.; Jan, J. S.; Teng, H. Synergistic combination of ether-linkage and polymer-in-salt for electrolytes with facile Li+ conducting and high stability in solid-state lithium batteries. Energy. Storage. Mater. 2024, 65, 103178.
19. Xie, K.; Fu, Q.; Chen, F.; et al. Controlling the supramolecular architecture enables high lithium cationic conductivity and high electrochemical stability for solid polymer electrolytes. Adv. Funct. Mater. 2024, 34, 2315495.
20. Zhang, D.; Shi, Y.; An, J.; Yang, S.; Li, B. Triallyl cyanurate copolymerization delivered nonflammable and fast ion conducting elastic polymer electrolytes. J. Mater. Chem. A. 2022, 10, 23095-102.
21. Zhang, L.; Xu, X.; Jiang, S.; et al. Halloysite nanotubes modified poly(vinylidenefluoride-co-hexafluoropropylene)-based polymer-in-salt electrolyte to achieve high-performance Li metal batteries. J. Colloid. Interface. Sci. 2023, 645, 45-54.
22. Cao, X.; Jia, H.; Xu, W.; Zhang, J. G. Review - localized high-concentration electrolytes for lithium batteries. J. Electrochem. Soc. 2021, 168, 010522.
23. Chen, J.; Li, Q.; Pollard, T. P.; Fan, X.; Borodin, O.; Wang, C. Electrolyte design for Li metal-free Li batteries. Mater. Today. 2020, 39, 118-26.
24. Selvanathan, V.; Halim, M. N. A.; Azzahari, A. D.; Rizwan, M.; Shahabudin, N.; Yahya, R. Effect of polar aprotic solvents on hydroxyethyl cellulose-based gel polymer electrolyte. Ionics 2018, 24, 1955-64.
25. Bradford, G.; Lopez, J.; Ruza, J.; et al. Chemistry-informed machine learning for polymer electrolyte discovery. ACS. Cent. Sci. 2023, 9, 206-16.
Cite This Article
How to Cite
Ma, Q.; Shen, S.; Lu, J.; Ai, L.; Hou, Y.; Li, Y.; Liu, J. Full-performance coordinated design for polymer-in-salt solid electrolyte. Energy Mater. 2025, 5, 500029. http://dx.doi.org/10.20517/energymater.2024.176
Download Citation
Export Citation File:
Type of Import
Tips on Downloading Citation
Citation Manager File Format
Type of Import
Direct Import: When the Direct Import option is selected (the default state), a dialogue box will give you the option to Save or Open the downloaded citation data. Choosing Open will either launch your citation manager or give you a choice of applications with which to use the metadata. The Save option saves the file locally for later use.
Indirect Import: When the Indirect Import option is selected, the metadata is displayed and may be copied and pasted as needed.
About This Article
Special Issue
Copyright
Data & Comments
Data
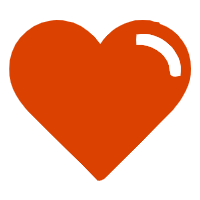
Comments
Comments must be written in English. Spam, offensive content, impersonation, and private information will not be permitted. If any comment is reported and identified as inappropriate content by OAE staff, the comment will be removed without notice. If you have any queries or need any help, please contact us at support@oaepublish.com.