Are AI and VR tools changing spine education and training?
Abstract
From diagnostics and treatments to surgical techniques and postoperative outcomes, the field of spine surgery is advancing at a historically unprecedented rate. Given the widespread integration of artificial intelligence (AI) in various industries, its implementation in the medical field is not a question of if, but when it will happen. AI’s ability to sort, analyze, and summarize vast quantities of data demonstrates great potential in assisting surgical professionals in all levels of training. Virtual reality (VR) enables users to explore and interact in a three-dimensional, computer-generated environment, and its application in the field of spine surgery can include bringing awareness and exposure of the field to medical students, surgical training and repetition of residents and fellows, and surgical planning for attendings. Augmented reality (AR) has significant potential through its versatile applications, offering benefits in medical education and training. While there are costs associated with the implementation of AI and VR in training curriculums for spine professionals, the long-term benefits and savings to various stakeholders outweigh the initial investment. This paper intends to offer a focused summary of the impact of AI and VR tools in spine education and training.
Keywords
INTRODUCTION
With the advancement of orthopedic procedures and the introduction of novel technologies, the education of medical students, residents, and fellows has become more comprehensive. Given the complexity of each subspecialty, there is a limited amount of training that can be allocated to a given field, and that is where artificial intelligence (AI) and virtual reality (VR) tools can be helpful if utilized properly. Spine pathologies are one of the leading disorders worldwide, with spine treatments constantly evolving and including an intricate network of instrumentation and graft materials. Providing residents and fellows with tools that assist in understanding and assessing spine pathologies and appropriate treatment options is critical for improving patient outcomes and enabling future spine clinicians to be part of innovation. While there are many advantages in implementing those technologies, the costs associated with implementation, as well as the allocation of resources and staff to carry out the initiatives, remain significant challenges across different institutions, independent of their size. While the initiation costs might be higher than traditional education modules[1], the overall cost of educating medical students, residents, fellows, and surgeons will be lower[2], potentially reducing the overall cost of patient care and benefiting various stakeholders[3].
AI
The authors of the AI textbook “Artificial Intelligence: A Modern Approach”, define AI as “the study of agents that receive percepts from the environment and perform actions”. They outline four key perspectives on AI: it can act humanly, think humanly, think rationally, and act rationally[4]. Additionally, there can be confusion between “artificial intelligence” and “machine learning”. Machine learning is a subfield of AI focused on enhancing performance through experience. While some AI systems rely on machine learning techniques to develop capabilities, others do not use these methods at all.
With its integration in nearly every industry, AI’s application in the medical field was inevitable. By analyzing an exponentially expanding quantity of electronic medical records, imaging, and patient-reported outcomes, AI is expected to assist healthcare professionals in predicting patients’ clinical outcomes quicker and with more accuracy than ever before. Consequently, AI is estimated to generate up to $150 billion in annual savings for the United States healthcare system by 2026[5]. AI is transforming the landscape of spine education and training, impacting all levels of medical professionals - from students and residents to practicing physicians. As the volume of medical knowledge and technological advancements continues to grow, AI tools are being integrated into educational frameworks and clinical practices. This integration not only aids medical students in mastering complex material but also supports residents in refining their surgical skills and helps practicing physicians enhance patient outcomes through improved preoperative decision making.
Education and training
The 21st century has transformed the medical field with new information, diagnostics, treatments, and surgical techniques being discovered, developed, and refined at a rate never seen before. Burdened with an overwhelming amount of new information while trying to build a foundation in medical knowledge, medical students are turning to a generative model of AI known as ChatGPT to optimize study time. ChatGPT is a large language model developed by OpenAI on November 30, 2002. Gathering a following of 100 million users within two months, it is the fastest-growing application to date[6]. With its ability to scan all available resources on the internet, ChatGPT can summarize and identify key points in seconds. By identifying key points and concepts, ChatGPT can generate study materials such as flashcards, practice questions, and self-assessments. As ChatGPT is interactive, the medical student can prompt it to further customize the materials and provide a personalized study plan. Additionally, ChatGPT has demonstrated its ability to simulate patient encounters, enabling the student to improve communication skills and practice clinical decision making[5].
As residents develop their surgical skills and increasingly feel pressure to maintain expertise in their education and training, AI can be combined with VR or augmented reality (AR) to provide immersive simulations. It can complement residency training by offering realistic and interactive surgical scenarios while providing automated, objective surgical performance feedback. Due to the delicate nature of the spine and the detrimental repercussions of injuring the spinal cord and nerve roots, this would be of great benefit for residents to practice and master techniques and approaches in spine surgery in a safe and controlled environment. Although not currently utilized, AI can assess their performance and identify what areas need improvement[7].
Surgical planning and outcomes
AI has demonstrated promising utility in assisting surgeons with the preoperative planning of spinal surgery, from diagnostics and risk stratification to the prediction of outcomes and complications. Currently, AI’s clinical use is limited to the generation of 3D models with vertebral body segmentation and labeling. Automated vertebral body detection and segmentation are widely available for both computed tomography (CT) and magnetic resonance imaging (MRI)[8]. Suzani et al. developed a type of AI called deep neural network that can detect and localize vertebrae in CT scans in less than 3 s with an accuracy of 96%[9]. Additionally, with the intention of further expanding AI’s application in spinal diagnostics, developments have been made in the identification of vertebral fractures, measuring of spine angles, evaluation of spinal cord pathology, screening assessment of osteoporosis, classification of degenerative changes, and detection of spinal metastases[10]. Several groups have used machine learning models in spinal deformity utilizing various radiological parameters to predict the severity of spinal deformity, spinal curvature, and spinopelvic parameters[11]. Advancements in AI in the identification of various spine and spinal cord pathologies would assist surgeons and healthcare professionals with earlier detection and a decrease in missed or delayed diagnosis.
With rising healthcare costs, there has been increased scrutiny of surgical outcomes, as many suspect them to be a large contributor. Therefore, greater emphasis has been placed on understanding the preoperative state of the patient, and the potential of AI predictive models in the preoperative risk stratification of patients can be profound. Currently, the American Society of Anesthesiologists (ASA) physical status classification system is the universally accepted method of determining the preoperative health of a patient. Kim et al. developed an algorithm that analyzed over 4,000 patients who underwent fusion for adult spinal deformities with predictive variables including sex, age, ethnicity, diabetes, smoking, steroid use, coagulopathy, functional status, ASA class > 3, body mass index (BMI), pulmonary comorbidities, and cardiac comorbidities. This algorithm outperformed ASA scoring in predicting every complication (P < 0.05), including cardiac complications, wound complications, venous thromboembolism, and mortality rates[12]. Furthermore, Arvind et al. trained various AI models to predict the same set of surgical complications following anterior cervical discectomy and fusion (ACDF) of more than 20,000 patients and found that they also outperformed ASA physical status classification (P < 0.05)[13]. By increasing the accuracy of preoperative risk stratification, clinicians would not only be better equipped to decide whether the postponement of surgery to optimize the patient is justifiable, but also be better prepared to deal with postoperative adverse events.
Limitations
AI in spine education and training is not without limitations. Firstly, some generative AI technologies, like ChatGPT, have been found to fabricate information and references, a behavior known as hallucination[6]. Moreover, AI relies on algorithms that require extensive data sets to extrapolate and deliver accurate, high-quality insights. However, the effectiveness of these models is fundamentally tied to the quality of the data used for training. Incomplete or inaccurate data will invalidate AI’s practicality. Additionally, a significant challenge to expanding AI’s application in spinal diagnostics is its robustness - an essential trait for enhancing diagnostic accuracy and improving patient outcomes. Developing reliable deep neural networks for machine learning is complex, as these systems must consistently and accurately analyze spinal images across a wide range of conditions and scenarios. Furthermore, although countless AI surgical applications are being developed, there is a paucity of literature investigating its adoption into clinical practice, especially in the field of spine surgery.
VR
Training and education of medical students, residents, surgeons, and patients
The rise of VR and its applications in the medical field has been remarkable, particularly in orthopedic surgery, where usage has surged by 300% over the past decade[14]. This growth is expected to continue, with further advancements and applications anticipated in the near future. VR can drastically transform medical training and education by enabling users to explore the human body and simulate conventional, new, or highly complex medical procedures through immersive virtual experience[15]. VR technology comes in various forms, with head-mounted devices (HMDs) being the most commonly used in medical settings, which have been shown to make learning content more accessible, engaging, and effective for acquiring better cognitive skills[16,17]. Barteit et al. conducted a systematic review to evaluate the effectiveness of VR HMDs in medical education, specifically for knowledge and skills training. One approach examined involved VR HMD scenarios where sensors were attached to real surgical instruments to navigate a virtually created operating room (OR). Participants reported that this training is beneficial to surgical approach learning[16].
Cadavers are commonly utilized in medical school as a method for medical students to become adept in human anatomy. However, there are limitations, as cadavers require skillful, laborious dissection to properly visualize anatomical structures. In some cases, these structures are obscured or inaccessible due to tissue damage caused during the dissection process. Furthermore, some schools may not have the means to replace the cadavers frequently, and students are required to interact with a cadaver that had been handled extensively already, leaving the structures poorly intact or completely unrecognizable. Time constraints and the need for cost-effectiveness have led medical schools to reduce the amount of time spent on anatomy and laboratory instruction using human cadaveric specimens[18]. VR tools may be effective solutions to those limitations as multiple studies have demonstrated their value in the education of medical students. Karbasi et al. reported that first-year medical students, who have less knowledge of human anatomy and experience with anatomical dissection, benefited from integrating VR into their curriculum[19]. Additionally, Cooper Medical School of Rowan University implemented an educational VR platform into the anatomy curriculum of first-year medical students and reported that students found it to be a valuable educational tool and even preferred it over traditional methods of learning anatomy[20]. Ekstrand et al.’ study provided evidence that immersive and interactive VR environments may provide effective supplemental tools for medical students learning the essentials of medical practice with the fact that the VR group presented significantly greater accuracy than the paper-based group, highlighting further needs for studies of VR as a learning tool in medical education[18].
Orthopedic physicians focus on diagnosing, treating, and repairing the musculoskeletal system, which requires proficiency in various surgical procedures. However, the level of exposure to these procedures can differ significantly among residency programs, potentially limiting residents’ skill development and affecting their performance as attending physicians. While VR is still in the early stages of adoption in orthopedic training, it offers an engaging platform for residents and physicians to gain realistic surgical experience across a wide range of procedures. Its human-machine interaction features varying levels of procedural complexity, creating an immersive learning pathway aimed at improving surgical performance and postoperative outcomes[15,21]. Studies by McCloskey et al. and Godzik et al. examined training systems used to teach pedicle screw placement and found a statistically significant reduction in penetration rates and improvement in accuracy rate in the VR groups compared to the control groups[2,22]. Hooper et al. conducted a study to assess the effectiveness of VR simulation for total hip arthroplasty (THA) in providing targeted surgical training to first-year orthopedic residents. They compared the improvement in specific aspects of surgical skills, surgical anatomy, and medical knowledge between the VR training group and the traditional cadaveric THA training group. The results indicated that the VR group showed greater improvement than the cadaveric group, although these differences were not statistically significant[23]. Logishetty et al. conducted a study involving a VR-trained group and a conventionally trained control group. The assessment was performed using a cadaveric THA, with the primary outcome based on the participants’ procedure-based assessment (PBA) scores. The VR group reported a significant score, which reflected procedural independence, in comparison to the control group[24]. The study authors reported that if VR training was conducted prior to live surgery, there was greater potential for a positive impact on technical skills. Berthold et al. conducted a systematic review of a study focused on pedicle screw placement with VR. The control group consisted only of attending physicians and showed a success rate of 82.9% for the VR-trained surgeons, compared to 74.2% for the control group, with statistical significance (P < 0.05). The VR group showed an improvement in their baseline from 69.2% to 82.9% after VR simulation training, suggesting that VR is an effective tool for improving attending physician skills[25]. One of the most important values in surgical skills is manual dexterity, which can be developed and perfected meticulously through repeated practice in VR simulation[23,26]. Immersive VR simulations are expected to evolve with advancements in software, enhancing procedural complexities and providing more training opportunities for both residents and attending surgeons. As a result, VR will become increasingly valuable as a tool for improving surgical skills[23]. Several studies have shown that VR in 3D neuroanatomical learning is significantly effective in improving neuroanatomical knowledge and retention. These improvements also extend to a better understanding of spatial relationships, resulting from both physical and virtual exposure to anatomy[26]. Additional benefits of VR simulation for training orthopedic surgeons include: minimal disposable costs, accessibility of consoles (i.e., one may practice anywhere, not just in a laboratory or OR), the ability of remote teaching and real-time feedback, availability of a wide range of simulations offering an assessment of objective skills, and the potential for skills learned to be translated to real patients[15,21,27].
Surgical planning
VR can supplement surgeons not just through simulation training but with preoperative surgical planning as well. In surgical planning or training, VR enables the exploration of various strategies, where individual steps can be undone or corrected, providing a safe environment for learning and refining techniques without the risk of real-world consequences[28]. In lieu of utilizing two-dimensional patient-specific radiological images, VR can reconstruct an interactive three-dimensional simulation of patients’ anatomy from their preoperative images, thus optimizing visualization of the patient’s anatomical structures and pathology[29]. VR technology has advanced to the point where it offers enhanced visualizations and interactive capabilities for planning complex patient cases, delivering faster and more efficient results than traditional desktop-based methods. Going beyond single-user applications, planning and collaborative VR has been employed to improve team collaboration, enabling seamless interaction between users, whether remotely or in the same physical location[28]. This would improve patient-specific preoperative planning and execution of a successful surgery with greater precision in dissection, alignment, and instrumentation[13]. Studies have reported that using VR systems for patient-specific preoperative planning leads to improved outcomes, including reductions in surgical time, damage to surrounding anatomy, bleeding, intraoperative fluoroscopy and radiation, and hospital stay[14,22,29].
Limitations to VR
While numerous studies demonstrate VR’s ability to enhance the knowledge and surgical performance of orthopedic residents and attendings, its integration into orthopedic training programs lags compared to other surgical specialties. Currently, VR training simulators are found in only a limited number of orthopedic residency programs and there is a lack of VR that supports the development of more complex orthopedic skills[21,27]. Additionally, the absence of physical sensation may reduce the precision required for surgical procedures, concerning some on its ability to be translated to real procedures. The lack of tissue responsiveness during incisions can be a challenge, especially in the spine with various surrounding tissues. Potential intraoperative complications that are caused by interactions of different instrumentation sets can present as a major limitation, especially in the early stages of training. Although participants in VR simulation training could be immersed in a realistic environment where they could repeat training processes multiple times, it did not diminish the reality that the simulators lack haptic feedback, tactility, pulsatile flow, and real-life simulation[30]. Furthermore, many VR tools rely on headsets, often causing dizziness for users[31]. Although VR systems excel in immersive surgical planning and training, their impact on surgical execution is limited because they do not allow surgeons to operate on patients using integrated, patient-specific information[29]. As with any new technology implementation, there are associated costs for hospitals and university departments. Although the cost of VR headsets has gone down, the software components needed for medical purposes remain expensive and often require additional revisions. The administrative and information technology costs are often forgotten when considering VR.
VR systems immerse users in a virtual world, thus limiting their view of reality and resulting in a lack of practicality in live surgeries[14]. AR creates an artificial layer over the real world, blending a virtual environment with the live, physical setting[2]. While VR creates an entirely artificial world that replaces the real one, AR integrates virtual elements into the real world, allowing users to remain connected to their physical environment. However, VR’s integration with AR would combine virtual and physical realities by superimposing digital imaging onto the surgical site while maintaining the ability to visualize anatomical landmarks during the surgical procedure[29]. By creating a visual overlay directly on the patient, an integrated VR and AR system exhibits great potential as a tool for preoperative planning and the training of medical students, residents, and surgeons in orthopedics procedures and spine surgeries[22,31].
AR
Education
AR is a tool capable of bridging artificial simulation and the real world by superimposing artificial images into the real environment while allowing its users to distinguish them[2]. AR can provide surgeons with a real-time three-dimensional view of patient anatomy without the need to move their field of vision from the patient during procedures, allowing for more accurate identification of trajectories and anatomy[1,32]. AR has significant potential through its versatile applications, offering benefits in medical education and training. Studies showed that the use of AR for medical education increased learning ability and provided a platform to increase practical skills in various areas[16], including significantly enhancing students’ understanding of anatomy. Due to its broad applications, AR has been integrated into medical education through various classroom study apps and clinical training simulators, helping to provide foundational medical knowledge. When used for technical repetition, AR allows students to practice surgical techniques in a realistic, simulated environment without the need for physical patients, thereby reducing potential risks and enabling safer, more effective skill development[33]. Once their skills have progressed through AR-based training, students can begin performing procedures on physical patients with greater confidence and competence, having already refined their techniques in a controlled, low-risk environment.
Training
VR platforms serve to provide rehearsal environments that are better suited for surgical execution. Its ability to serve as a training platform for technical skills and repetitive learning has been shown to improve residents’ abilities, resulting in increased accuracy through repeated practice. Luciano et al. evaluated the use of an AR simulation for thoracic pedicle screw placement with real patient CT where they observed a 15% mean improvement in accuracy with a 50% reduction in standard deviation from practice to testing[34]. AR is capable of enhancing learning experiences, improving procedural accuracy, and providing immersive, hands-on training opportunities for medical professionals. AR’s clinical applications are found to be much broader than VR[2]. An AR application designed to train physicians with a step-by-step guide in rod bending and implantation showed a significant reduction in rod bending errors compared to the traditional free-hand method[35]. The study demonstrated that AR could enhance precision and improve the accuracy of surgical techniques. AR has the capability to decrease the learning curve for complex spine procedures and promote surgical skills, encouraging confidence in physician spine surgeons and residents.
CONCLUSION
AI’s ability to analyze, predict, and generate large quantities of data could optimize and customize medical students’ time and study materials, enhance and assess resident performance, and assist surgeons in optimizing preoperative planning. VR provides an immersive simulation capable of displaying a three-dimensional, computer-generated environment that could enable trainees and surgeons to visualize and interact with human anatomy and surgical procedures. AR can project patient CT or artificial images into the real world while keeping the distinction between the simulation and the real world. It enhances skills, decreases the learning curve, and promotes accuracy. It is more clinically adapted in surgery than VR. VR and AR differ in the sense that VR provides a new artificial world, replacing the real world, while AR overlays digital elements onto the real world without separating the user from reality. AR and VR can be combined, bringing greater potential for their use in medical education, benefiting medical students and the training of residents and physicians. Additionally, the integration of AI with VR can provide personalized feedback during simulations. Although the current state of AI and VR integration into spine education and training is preliminary, they demonstrate promising potential and may be essential in supporting surgical professionals at every stage of their training to adapt to a rapidly evolving field.
DECLARATIONS
Authors’ contributions
Idea generation, literature search, writing, and revision: Baker M, Lontchi R
Idea generation, writing, revision, and supervision: Buser Z
Availability of data and materials
Not applicable.
Financial support and sponsorship
None.
Conflicts of interest
Buser Z: Grants/Research support: Medical Metrics (past, paid directly to institution/employer); AO Spine (past, paid directly to institution/employer); Nexus Spine (past, paid directly to institution/employer), MiMedx (past, paid directly to institution/employer), SBIR NIH (past, Paid directly to institution/employer); Next Science (past, paid directly to institution/employer); Consultancy: Next Science (paid directly to institution/employer). Scientific Advisory Board: Medtronic, Medical Metrics, DePuy Synthes. Trips/Travel: AO Spine, NASS (travel reimbursement for meetings); Board of Directors: LSRS (Nonfinancial, Co-chair Program Committee); Committees: AO Spine Knowledge Forum Degenerative (Steering Committee Member); North American Spine Society: Research Project Management Committee (Vice chair), Section on Biologics & Basic Research (Co-chair), co-chair of Section on Innovative Spine Research and Novel Technologies, Coverage Committee and The Spine Journal Peer Reviewers (Committee member). Patents (issued): Biomarkers for painful intervertebral discs and methods of use thereof. The other authors declared that there are no conflicts of interest.
Ethical approval and consent to participate
Not applicable.
Consent for publication
Not applicable.
Copyright
© The Author(s) 2025.
REFERENCES
1. Hasan S, Miller A, Higginbotham D, Saleh ES, McCarty S. Virtual and augmented reality in spine surgery: an era of immersive healthcare. Cureus. 2023;15:e43964.
2. Godzik J, Farber SH, Urakov T, et al. “Disruptive technology” in spine surgery and education: virtual and augmented reality. Oper Neurosurg. 2021;21:S85-93.
3. Sahni N, Stein G, Zemmel R, Cutler D. The potential impact of artificial intelligence on health care spending. In: Agrawal A, Gans J, Goldfarb A, Tucker C, editors. The economics of artificial intelligence: health care challenges Chicago: University of Chicago Press. 2023. pp. 49-86.
4. Russell SJ, Norvig P. Artificial intelligence: a modern approach. 4th edition. Pearson. Available from: http://lib.ysu.am/disciplines_bk/efdd4d1d4c2087fe1cbe03d9ced67f34.pdf. [Last accessed on 3 Jan 2025].
5. Lisacek-Kiosoglous AB, Powling AS, Fontalis A, Gabr A, Mazomenos E, Haddad FS. Artificial intelligence in orthopaedic surgery. Bone Joint Res. 2023;12:447-54.
6. Eysenbach G. The role of ChatGPT, generative language models, and artificial intelligence in medical education: a conversation with ChatGPT and a call for papers. JMIR Med Educ. 2023;9:e46885.
7. Guerrero DT, Asaad M, Rajesh A, Hassan A, Butler CE. Advancing surgical education: the use of artificial intelligence in surgical training. Am Surg. 2023;89:49-54.
8. Štern D, Vrtovec T, Pernuš F, Boštjan Likar B. Automated determination of the centers of vertebral bodies and intervertebral discs in CT and MR lumbar spine images. In: Medical Imaging 2010: Image Processing. 2010. pp. 1550-61.
9. Suzani A, Seitel A, Liu Y, Fels S, Rohling RN, Abolmaesumi P. Fast automatic vertebrae detection and localization in pathological CT scans - a deep learning approach. In: Navab N, Hornegger J, Wells WM, Frangi AF, editors. Medical Image Computing and Computer-Assisted Intervention - MICCAI 2015. Cham: Springer International Publishing; 2015. pp. 678-86.
10. Martín-Noguerol T, Oñate Miranda M, Amrhein TJ, et al. The role of artificial intelligence in the assessment of the spine and spinal cord. Eur J Radiol. 2023;161:110726.
11. Suryavanshi J, Foley D, Mccarthy MH. Artificial intelligence in spinal deformity. J Orthop Rep. 2025;4:100358.
12. Kim JS, Merrill RK, Arvind V, et al. Examining the ability of artificial neural networks machine learning models to accurately predict complications following posterior lumbar spine fusion. Spine. 2018;43:853-60.
13. Arvind V, Kim JS, Oermann EK, Kaji D, Cho SK. Predicting surgical complications in adult patients undergoing anterior cervical discectomy and fusion using machine learning. Neurospine. 2018;15:329-37.
14. Combalia A, Sanchez-Vives MV, Donegan T. Immersive virtual reality in orthopaedics-a narrative review. Int Orthop. 2024;48:21-30.
15. Luca A, Giorgino R, Gesualdo L, et al. Innovative educational pathways in spine surgery: advanced virtual reality-based training. World Neurosurg. 2020;140:674-80.
16. Barteit S, Lanfermann L, Bärnighausen T, Neuhann F, Beiersmann C. Augmented, mixed, and virtual reality-based head-mounted devices for medical education: systematic review. JMIR Serious Games. 2021;9:e29080.
17. Haowen J, Vimalesvaran S, Myint Kyaw B, Tudor Car L. Virtual reality in medical students’ education: a scoping review protocol. BMJ Open. 2021;11:e046986.
18. Ekstrand C, Jamal A, Nguyen R, Kudryk A, Mann J, Mendez I. Immersive and interactive virtual reality to improve learning and retention of neuroanatomy in medical students: a randomized controlled study. CMAJ Open. 2018;6:E103-9.
19. Karbasi Z, Niakan Kalhori SR. Application and evaluation of virtual technologies for anatomy education to medical students: a review. Med J Islam Repub Iran. 2020;34:163.
20. Kolla S, Elgawly M, Gaughan JP, Goldman E. Medical student perception of a virtual reality training module for anatomy education. Med Sci Educ. 2020;30:1201-10.
21. Schöbel T, Schuschke L, Youssef Y, Rotzoll D, Theopold J, Osterhoff G. Immersive virtual reality in orthopedic surgery as elective subject for medical students: first experiences in curricular teaching. Orthopadie. 2024;53:369-78.
22. McCloskey K, Turlip R, Ahmad HS, Ghenbot YG, Chauhan D, Yoon JW. Virtual and augmented reality in spine surgery: a systematic review. World Neurosurg. 2023;173:96-107.
23. Hooper J, Tsiridis E, Feng JE, et al; NYU Virtual Reality Consortium. Virtual reality simulation facilitates resident training in total hip arthroplasty: a randomized controlled trial. J Arthroplasty 2019;34:2278-83.
24. Logishetty K, Rudran B, Cobb JP. Virtual reality training improves trainee performance in total hip arthroplasty: a randomized controlled trial. Bone Joint J. 2019;101-B:1585-92.
25. Berthold DP, Muench LN, Rupp MC, et al. Head-mounted display virtual reality is effective in orthopaedic training: a systematic review. Arthrosc Sports Med Rehabil. 2022;4:e1843-9.
26. Vayssiere P, Constanthin PE, Herbelin B, Blanke O, Schaller K, Bijlenga P. Application of virtual reality in neurosurgery: patient missing. A systematic review. J Clin Neurosci. 2022;95:55-62.
27. Cate G, Barnes J, Cherney S, et al. Current status of virtual reality simulation education for orthopedic residents: the need for a change in focus. Global Surg Educ. 2023;2:46.
28. Allgaier M, Chheang V, Saalfeld P, et al. A comparison of input devices for precise interaction tasks in VR-based surgical planning and training. Comput Biol Med. 2022;145:105429.
29. van der Kruk SR, Zielinski R, MacDougall H, Hughes-Barton D, Gunn KM. Virtual reality as a patient education tool in healthcare: a scoping review. Patient Educ Couns. 2022;105:1928-42.
30. Joseph FJ, Vanluchene HER, Bervini D. Simulation training approaches in intracranial aneurysm surgery-a systematic review. Neurosurg Rev. 2023;46:101.
31. Ghaednia H, Fourman MS, Lans A, et al. Augmented and virtual reality in spine surgery, current applications and future potentials. Spine J. 2021;21:1617-25.
32. Yoo JS, Patel DS, Hrynewycz NM, Brundage TS, Singh K. The utility of virtual reality and augmented reality in spine surgery. Ann Transl Med. 2019;7:S171.
33. Yuk FJ, Maragkos GA, Sato K, Steinberger J. Current innovation in virtual and augmented reality in spine surgery. Ann Transl Med. 2021;9:94.
34. Luciano CJ, Banerjee PP, Bellotte B, et al. Learning retention of thoracic pedicle screw placement using a high-resolution augmented reality simulator with haptic feedback. Neurosurgery. 2011;69:ons14-9; discussion ons19.
Cite This Article

How to Cite
Download Citation
Export Citation File:
Type of Import
Tips on Downloading Citation
Citation Manager File Format
Type of Import
Direct Import: When the Direct Import option is selected (the default state), a dialogue box will give you the option to Save or Open the downloaded citation data. Choosing Open will either launch your citation manager or give you a choice of applications with which to use the metadata. The Save option saves the file locally for later use.
Indirect Import: When the Indirect Import option is selected, the metadata is displayed and may be copied and pasted as needed.
About This Article
Special Issue
Copyright
Data & Comments
Data
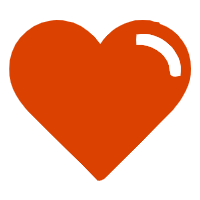
Comments
Comments must be written in English. Spam, offensive content, impersonation, and private information will not be permitted. If any comment is reported and identified as inappropriate content by OAE staff, the comment will be removed without notice. If you have any queries or need any help, please contact us at [email protected].