Contribution of 3D virtual modeling in locating hepatic metastases, particularly “vanishing tumors”: a pilot study
Abstract
Aim: This article aims to explore how three dimensions (3D) virtual modeling enhances accuracy and efficiency in detecting hepatic metastases, with a specific focus on “vanishing tumors” that are difficult to detect using traditional imaging techniques. It also aims to demonstrate the potential impact of these advanced technologies on improving diagnostic and treatment strategies for patients with liver cancer.
Methods: Eight patients with liver metastases from colorectal cancer were studied using magnetic resonance imaging (MRI) and 3D virtual modeling to enhance surgical planning by accurately locating lesions. The concordance between these imaging techniques and the Gold Standard was assessed using Gwet’s AC1 coefficient, with statistical analysis performed through resampling methods and non-parametric tests due to non-normal AC1 distribution. Liver segmentation was conducted semi-automatically using SurgiPrint software, which is crucial for detecting lesions undetectable post-chemotherapy. The study’s evaluation model involved questionnaires for medical professionals across four cohorts, aiming to determine the 3D model’s effectiveness in identifying lesion locations for surgery.
Results: This study investigated the efficacy of 3D virtual modeling in identifying hepatic metastases, particularly comparing its accuracy with traditional MRI in locating lesions. The findings indicate that MRI generally provided better concordance with the Gold Standard for lesion localization, except for a few experienced users who had prior familiarity with the 3D models. Despite the mixed results in accuracy, the study suggests potential benefits of 3D modeling in enhancing surgical planning and execution, particularly in detecting “vanishing” liver metastases that are difficult to visualize with standard imaging techniques. The research aligns with broader evidence indicating the utility of 3D models in improving outcomes in hepatic surgery by enabling more precise resections and reducing postoperative complications. However, the study also notes the challenge of quantifying the added value of 3D modeling due to the unique nature of each surgical case and the potential bias in the user experience with the technology.
Conclusion: The conclusion of this study underscores the significant potential of 3D virtual modeling in enhancing the precision of locating and resecting hepatic metastases, particularly those elusive “vanishing tumors”. This research underscores the value of integrating advanced 3D imaging techniques into surgical practice, suggesting a paradigm shift toward more accurate and less invasive liver surgeries. Future studies should aim to further quantify the benefits of 3D modeling in surgical outcomes, investigate its utility across different surgical experiences, and explore the integration of artificial intelligence (AI) to maximize its effectiveness in clinical settings.
Keywords
INTRODUCTION
Colorectal cancer is the third most frequently diagnosed cancer in men and the second in women, with more than 1,800,000 new cases and almost 850,000 deaths per year worldwide (WHO). The incidence and mortality rates vary considerably from country to country[1,2] and this disease is increasingly affecting younger patients[3]. Hepatic metastases from colorectal cancers (CRLM) are present in 15% to 20% of patients at the time of diagnosis, and 20% to 25% of non-metastatic patients will develop CRLM within three years following the discovery of their colorectal cancer[4,5].
The liver is the most common site for metastases, accounting for 70% of cases[6]. Liver resection is acknowledged as the sole treatment option with the potential to cure metastatic colorectal cancer in the liver.
In the realm of liver surgery, diagnostic images obtained from pre-surgical computed tomography (CT) scans and magnetic resonance imagings (MRIs) are crucial in shaping the strategy for treatment. These images are essential in devising and directing the surgical procedure, particularly when aiming to conserve liver tissue during resections. While these medical images are typically viewed in a two-dimensional (2D) format, it is imperative for surgeons to interpret and visualize these images in three dimensions (3D) to grasp a comprehensive understanding of the anatomical layout.
Prior to mapping out the surgical intervention for the liver, it is imperative for surgeons to pinpoint the tumor’s location by determining which segment of the liver is affected. The classification of these liver segments traditionally follows the well-established Couinaud system, a method commonly recognized and instructed in medical education[7]. Identifying the affected segment is achieved by meticulously examining the various layers of the scan and/or MRI images, using the hepatic vessels as reference points to demarcate the liver into distinct segments. This process demands a significant ability to perceive spatial relationships and often involves a considerable amount of time, particularly with complex cases or when the liver’s structure deviates from the usual anatomy.
The project was initiated in this context. It aims to evaluate the real benefit of 3D virtual liver models in the surgery of colorectal cancer liver metastasis resection.
Therefore, we decided to create 3D virtual models [Figure 1] in patients who had undergone cross-sectional imaging for surgical resection. The 3D virtual model is displayed on the left screen in the photo. The metastases are in green. The portal vein is in light blue, and the suprahepatic veins and the inferior vena cava are in dark blue.
Figure 1. Photo showing a radiologist’s console with 2D MRIs of a patient with liver metastases and his 3D virtual model. 2D: Two-dimensional; MRIs: magnetic resonance imagings; 3D: three dimensions.
The purpose of the 3D virtual model is to help the surgeon understand the exact location of the lesions and their relationship to the hepatic vessels.
The aim of this research is to investigate if employing a 3D virtual representation enhances the comprehension of the liver’s spatial configuration, and specifically of the segments affected by metastatic lesions, compared to the conventional method, namely the use of 2D images only.
The use of 3D virtual models could also be extended to other indications, such as liver transplants for patients with multiple liver metastases.
The article by Brandi et al. (2020) discusses the feasibility of post-transplant chemotherapy for patients who have undergone liver transplantation for colorectal cancer liver metastases[8]. The main focus is to assess whether chemotherapy following a liver transplant is a viable treatment option in such cases. This type of study could be coupled with the use of virtual 3D models to help surgeons use 3D models before liver transplantation. This could be another subject for future studies.
MATERIAL AND METHODS
Statistics
The AC1 coefficient by Gwet and its 95% confidence interval (CI) were calculated to assess the concordance between “location” measurements (by reader and by technique) and the Gold Standard. The strength of the concordance was interpreted as follows: weak: AC1 0.2, medium: 0.2 < AC1 ≤ 0.4, moderate: 0.4 < AC1 ≤ 0.6, good: 0.6 < AC1 ≤ 0.8, and very good: AC1 > 0.8.
The difference in AC1 between MRI and 3D was assessed using a resampling procedure (without replacement) based on 200 samples of N = 41 patients randomly drawn from the total cohort of N = 56 lesions. As the AC1 distributions were not normal according to the Shapiro-Wilk test, a Wilcoxon test was performed, from which the mean difference in AC1 was reported.
A P-value < 0.05 was considered statistically significant for all tests performed. The analyses were carried out using Statsdirect software v3.3.5 and Matlab software R2021b.
Comments
1. AC1 value ranges for concordance:
- Weak: AC1 ≤ 0.2
- Medium: 0.2 < AC1 ≤ 0.4
- Moderate: 0.4 < AC1 ≤ 0.6
- Good: 0.6 < AC1 ≤ 0.8
- Very good: AC1 > 0.8
2. Target AC1 value for a successful study:
The study aims to achieve an AC1 value greater than 0.8 to be considered successful, indicating a “very good” level of concordance.
3. Explanation of AC1 > 0.8:
An AC1 value greater than 0.8 indicates a very good concordance, meaning that the measurements being compared (e.g., between a reader, a technique, and the Gold Standard) are highly consistent. This suggests a high level of accuracy in the study’s results.
Patient selection
We selected 8 patients with hepatic metastases from primary colorectal cancer who had undergone diagnostic imaging (MRI) and a 3D virtual model before surgery. In this case, each patient underwent hepatic MRI as part of their hepatic extension assessment.
The sequences used were: T1Dixon before and after Gadolinium injection (Dotarem) - DWI/ADC - T2FS.
The MRI assessment served to document the position of the lesions and identify in which segment each lesion is located. The position of the lesions was used by the surgeon to organize their surgical planning.
This positioning is crucial and determines the surgical strategy. Indeed, from a simple wedge resection, the surgical procedure can change to a segmentectomy or even a lobectomy if the lesions are bilobar or located in the same segment.
After documenting each lesion of each patient (56 lesions spread over 8 patients), we proceeded with
Liver segmentation
We used the SurgiPrint software (www.surgiprint.com).
Hepatic segmentation was performed using a semi-automatic method thanks to pre-installed modalities on the SurgiPrint software. The hepatic tissue, as well as the portal and suprahepatic vessels, were preselected by the program. A brush tool that works by expanding tissue based on the ROI was used to draw and correct the contours of the liver, the portal vein and its branches, as well as the suprahepatic veins and the inferior vena cava.
This software was developed and designed to segment hepatic vessels based on tomographic imaging.
The use of SurgiPrint software offered us numerous advantages.
The first and greatest was the ability to model lesions not visible on per-operative ultrasound or on the CT scanner. When a patient had undergone neoadjuvant chemotherapy, their lesions may regress and no longer be visible except on MRI.
We know that there are tumor cells visible on histology. These lesions are called DLM: “Disappear Liver Metastasis”. These are lesions that have melted under chemotherapy but with a tumor residue visible only on diffusion sequences. Indeed, these lesions present a diffusion restriction due to their hypercellularity.
Our surgeons sometimes reported that these lesions are not visible on per-operative ultrasound either. Therefore, the 3D virtual modeling is of great utility to them.
The resection specimen was sent to pathology, and we obtained control and confirmation that these wedges are the site of residual metastatic lesion.
3D model production
Once segmentation was obtained, each element was exported separately as a stereolithographic file (.STL), which can also be used for 3D printing or virtual reality (VR). All the virtual models are accessible on Android and/or iOS with a simple internet connection [Figure 2].
Figure 2. 3D virtual model of a patient with liver metastasis. In dark blue, the suprahepatic veins and inferior vena cava. In light blue, the portal vein and in green, the tumors. 3D: Three dimensions.
The anonymity of each patient was preserved to comply with GDPR rules.
The approximate time taken to create a 3D virtual model is 24 to 48 h. The approximate time to create a printed model is 10 days.
The production cost for a 3D model is around $700.
Future efforts and progress should focus on reducing both time and cost, paving the way for more efficient and accessible solutions.
Continuous advancements in technology are being made to reduce both the time required to create 3D models and their associated costs. These developments are expected to improve the efficiency of 3D modeling and make the process more accessible for routine use in clinical settings.
The long-term objective is, therefore, to create software that will automate the creation of these 3D models in just a few minutes.
Methods
Evaluation Model:
Our goal is to assess the added value of the 3D virtual model for the localization of hepatic lesions and to be able to specify in which segment the lesion is located.
The test consists of two questionnaires.
We divided the participants into four cohorts.
1. Senior Hepatic Surgeon
2. Senior MACS in Surgery (referred to as junior surgeons).
3. Senior Abdominal Radiologist
4. Senior MACS in Radiology (referred to as junior radiologists).
Each cohort consists of three participants.
For each lesion, participants had to determine which segment it was located, based either on the MRI or on the 3D virtual models [Figure 3].
The Gold Standard was performed by a consortium of three radiologists.
RESULTS
The results obtained were analyzed with great care by our statistician. Here are the findings [Table 1]:
Each participant receives three scores. The first two are the result of positioning the lesions based on the MRI and then on the 3D virtual model. These first two scores are each out of a total of 56 points. When the two scores are added together, the total is out of 112.
Ranking of participants and their scores
IRM | 3D | Total sur 112 |
P7 (51) | P1 (44) | P5 (88) |
P8 (50) | P6 (43) | P1/P3/P9/P10 (87) |
P3 et P10 (49) | P5 (41) | P7 (85) |
P9(48) | P9 (39) | P8 (84) |
P5 et P12 (47) | P3 et P10 (38) | P6 et P12 (83) |
P11 (44) | P12 (36) | P4 (78) |
P1 et P4 (43) | P4 (35) | P11 (77) |
P2 et P6 4(0) | P7 P8 et P2 (34) | P2 (74) |
P11 (33) |
Senior surgeon:
Participant 1: 43/56 (IRM) + 44/56 (3D) = 87/112
Participant 2: 40 (IRM) + 34 (3D) = 74
Participant 3: 49 (IRM) 38 (3D) = 87
Junior surgeon:
Participant 4: 43 (IRM) + 35 (3D) = 78
Participant 5: 47 (IRM) + 41 (3D) = 88
Participant 6: 40 (IRM) + 43 (3D) = 83
Senior radiologist:
Participant 7: 51 (IRM) + 34 (3D) = 85
Participant 8: 50 (IRM) + 34 (3D) = 84
Participant 9: 48 (IRM) + 39 (3D) = 87
Junior radiologist:
Participant 10: 49 (IRM) + 38 (3D) = 87
Participant 11: 44 (IRM) + 33 (3D) = 77
Participant 12: 47 (IRM) + 36 (3D) = 83
Agreement with the Gold Standard
The concordance between individual “location” measurements and the Gold Standard is presented in Table 2. With MRI images, concordance ranges from poorer (all junior readers and senior surgeons) to very good (all senior radiologists). Using the 3D virtual model, concordance ranges from moderate to good, regardless of the experience or category the readers belong to.
Agreement with the Gold Standard on the location of the lesion
Rad junior | Rad senior | Surg junior | Surg senior | |
MRI | 0.83 [0.73;0.94] | 0.9 [0.81;0.98] | 0.73 [0.6;0.9] | 0.74 [0.61;0.86]* |
0.73 [0.69;0.86] | 0.88 [0.78;0.97] | 0.83 [0.73;0.94] | 0.64 [0.53;0.81] | |
0.81 [0.7;0.93] | 0.82 [0.71;0.93]* | 0.79 [0.68;0.91] | 0.86 [0.76;0.96]* | |
3D | 0.63 [0.48;0.77] | 0.54 [0.39;0.69] | 0.56 [0.42;0.71] | 0.73 [0.6;0.86] |
0.52 [0.37;0.67] | 0.54 [0.4;0.69] | 0.68 [0.54;0.81]* | 0.55 [0.41;0.7]* | |
0.58 [0.44;0.73] | 0.61 [0.47;0.76]* | 0.73 [0.6;0.86] | 0.633 [0.5;0.77] |
Overall, all readers located the lesions more accurately based on the MRI except for one senior surgeon Dr. Coubeau and his assistant Dr. de Hemptinne, who both used the models several times before the test.
Difference in concordance
The results of the resampling procedure are presented in Table 3. All readers show significantly better concordance with the Gold Standard when using MRI images than when using the 3D model (all P-values < 0.05 except for one reader). The differences in AC1 range from +0.06 (junior surgeon) to +0.35 (senior radiologist) in favor of the MRI.
Difference in agreement between MRI and 3D
P-value | |||
Rad junior | Rad senior | Surg junior | Surg senior |
+0.2 [+0.2;+0.21] | +0.35 [+0.34;+0.35] | +0.17 [+0.16;+0.17] | - |
< 0.0001 | < 0.0001 | < 0.0001 | 0.1153 |
+0.2 [+0.2;+0.21] | +0.32 [+0.31;+0.33] | +0.16 [+0.15;+0.16] | +0.12 [+0.11;+0.12] |
< 0.0001 | < 0.0001 | < 0.0001 | < 0.0001 |
+0.2 [+0.23;+0.24] | +0.21 [+0.19;+0.21] | +0.06 [+0.06;+0.07] | +0.23 [+0.22;+0.23] |
< 0.0001 | < 0.0001 | < 0.0001 | < 0.0001 |
Analysis of discrepancies
Five readers measure at least one “location = 1”, while this location is absent from the Gold Standard.
DISCUSSION
The development of 3D modeling has expanded across various healthcare fields, notably in orthopedics and maxillofacial surgery. Yet, it is less commonly adopted in routine general and abdominal surgery[9]. There is a growing body of literature on the subject, but few studies involve large cohorts[9,10]. Recent evidence has shown that the use of 3D virtual models in hepatic surgeries reduces operative risks and increases the success rate in resecting hepatic metastases and hepatocellular carcinomas[11].
At present, surgeons depend on pre-surgical imaging techniques like CT scans and/or MRIs for making clinical judgments, in addition to formulating and navigating surgical procedures. These images, while capturing volume, are displayed in a 2D format and must be conceptually transformed into 3D to enhance the perception of the spatial orientation of lesions and blood vessels [Figure 4].
Figure 4. Visualization of a sketch showing the surgeon’s surgical strategy for a patient with liver metastases.
Liver surgery remains the cornerstone of managing these patients, but less than 25% of them are initially resectable[12]. The response to current chemotherapies has significantly increased resectability by reducing hepatic tumor volume. Hence, medical and surgical advances in recent years have changed the management of patients with colorectal cancer and hepatic metastases. After resecting colorectal liver metastases, the risk of recurrence remains high (50%-80%), with the liver being the primary site of recurrence in half of the cases[3,4]. The surgical principle, therefore, is to apply the most selective actions possible, preserving as much healthy parenchyma as possible (Parenchyma Sparing). This approach involves detailed planning of the surgical procedure. Typically, the surgical team relies on 2D images from CT scans and MRI acquisitions. This can also be done by machines using Syngovia[13], but there are still many errors, and the software is not yet 100% accurate.
Segmentation of medical imagery involves individually delineating various anatomical features for examination and subsequent conversion into three-dimensional models. This segmentation necessitates the use of dedicated software that processes volumetric information. The segmentation could be manual, necessitating extensive user involvement; semi-automated, with minimal user input; or completely automated, requiring no user interaction. The segmented images are then transferred to PACS systems as a series of images with movement constrained to specific pre-set angles of view or paths of rotation. This restriction might pose a hindrance if the viewing angle needed by the surgeon is not pre-configured[14]. Conversely, a virtual 3D model can be moved, rotated, and viewed from endless perspectives in the operating room, offering total freedom of use to the user [Figure 5].
Figure 5. Surgeon operating on a patient with liver metastases using intraoperative ultrasound and the vital 3D model. 3D: Three dimensions.
The use of multiplanar reconstructions allows for planning the surgical procedure by enabling clear visualization of lesions and their spatial relations with vascular and biliary structures. This virtual vision remains complex to appreciate despite the use of computerized three-dimensional models.
SurgiPrint and the hepatobiliary surgery team at the Cliniques Universitaires Saint-Luc have decided to develop 3D virtual liver models and evaluate their use in the operating room for patients with hepatic metastases undergoing surgical resection.
We, therefore, selected patients with metastatic colorectal liver disease: the extrapolated object from standard imaging examinations is virtually modeled in three dimensions and used perioperatively to improve the spatial visualization of tumors relative to vascular structures.
The main added value of using 3D virtual models is to enable a more precise resection of hepatic lesions, thus sparing healthy hepatic parenchyma during surgery[12].
Parenchyma-sparing hepatic resection is as safe and effective as traditional anatomical resection[12]. The parenchyma-sparing surgical approach thus reduces the risk of postoperative hepatic complications and liver insufficiency, facilitating repeated resections in the event of recurrence[15].
A newly released study, deriving from a randomized controlled trial, corroborates that patients receiving liver resections that conserve parenchyma for colorectal metastases experience a substantial decrease in post-surgical complications, reduced duration of hospitalization, and improved cost-efficiency compared to traditional open surgical procedures[16].
Intrinsically, it is very difficult to prove an objective and quantifiable added value of a 3D model. Indeed, each operation is unique, metastases are never positioned the same way, and one would need to operate on each patient twice under the same conditions (once with the model and once without), which is obviously impossible.
We, therefore, decided to verify if the 3D virtual model helps to better localize lesions in the liver and to assert in which liver segment the lesion is located. This approach thus allows for a more objective verification of whether or not lesions are better spotted and located thanks to the 3D virtual model.
The goal is to evaluate the contribution of the virtual object in locating hepatic lesions (identification of hepatic segments associated with lesions) and if the 3D virtual model actually improves the planning and surgical exploration of hepatic metastases.
Our second question concerns whether there is a difference in outcomes based on cohorts. It is worth considering if 3D modeling is more beneficial to surgeons or radiologists and whether there is variability based on their personal experiences.
The results are quite intriguing. Among the 12 candidates who participated in the questionnaire, only 2 achieved a better result regarding the positioning of lesions in the 3D category. Interestingly, these were not random candidates but the senior surgeon who had used each of the models in the operating room, as well as his surgical assistant, who also used the model and was present in the operating room in most cases.
This suggests that 3D may be more useful to certain surgeons, but there appears to be a “teaching” bias in the use of these models. Indeed, all models used for the questionnaire had previously been used by Dr. Coubeau, who had ample time to analyze them for many minutes, even hours, before the operation, in the operating room, and even after the surgery [Figure 5]. The same applies to his assistant, who attended almost all the surgeries.
This supports the notion of a probable teaching bias, as only two surgeons achieved a better score, not the entire group of surgeons.
Drs. Coubeau and de Hemptinne likely had time to use the models, handle them, and understand their positioning in space.
Beyond our questionnaire, which was submitted to a dozen candidates, we observed other benefits and questioned additional contributions of 3D segmentation.
Indeed, we objectively evaluated only one criterion in this thesis, namely the positioning of lesions. However, throughout our nearly 2-year research, we observed other unexpected benefits and objectives.
According to anatomopathological analysis, the rate of resection of all metastases was in healthy margins and, therefore, complete. The next objective will be to use statistical analyses to compare the healthy margin resection rate of liver metastases with and without the use of 3D virtual models.
For example, one of the secondary objectives we could evaluate is the contribution of a “super-review” of 2D images necessary for creating the virtual 3D model, for refining radiological diagnosis (localization of lesions and vascular relationships). It is not uncommon to read in some hepatic MRI reports: “Bilobar hepatic lesions, the largest measuring”.
However, in reality, when modeling these MRIs in 3D, we must meticulously examine each slice of every sequence to achieve conclusive results for use by the surgeon in the operating room. We have even discovered additional metastases. The most compelling example involves what are called “DLM” or disappearing liver metastases.
The 3D modeling process also allows for the “geographic” resection of lesions sterilized by chemotherapy (DLM or disappearing liver metastases).
Metastatic lesions known as DLMs are those no longer found on the CT scanner or on the injected phases of MRI but still show a subtle restriction on certain sequences. We, therefore, know that tumor residue persists at this level and thus also include these lesions in our virtual 3D model.
Indeed, it is sometimes difficult in routine practice to detect “DLMs”[17]. Most of the time, when patients undergo MRI to monitor the progression of their disease, they have already received neoadjuvant therapy. The clinician then wonders about the state of the hepatic parenchyma and the evolution of hepatic metastases.
Some tumors may have significantly diminished following chemotherapy, making them challenging to identify via ultrasound or MRI. To locate any remaining tumors, we adhere to a specific protocol involving T1 Dixon imaging both before and after the administration of Gadolinium in multiple phases, diffusion sequences with an ADC map, and T2 Fat-SAT sequences.
The expertise of the radiologist is vital in such instances as they are tasked with the detection of these minute lesions that may only be evident on a single imaging sequence.
There are instances where metastatic lesions are not observable on T1 Dixon imaging, making their visualization exceedingly difficult.
Occasionally, diffusion sequences are the sole means by which radiologists can identify lingering tumor lesions. Particularly at high “b” values, these “disappearing lesions” that are undetectable on other sequences can still exhibit diffusion restriction, indicating the presence of residual tumor cells.
Furthermore, the field of radiology and hepatobiliary surgery is advancing, and radiologists are increasingly expected to be proficient in using artificial intelligence (AI) tools, such as 3D reconstruction technologies.
It is no longer sufficient for radiologists to merely note the presence of a residual tumor in a certain liver segment in their reports. They must also be equipped to aid surgeons in the planning of their operations by using 3D modeling to pinpoint the position of the residual lesion, which may only be visible on diffusion sequences, in relation to surrounding vessels. This allows surgeons in the operating theater to navigate more effectively during the procedure to excise the lesion. This is crucial because even with perioperative ultrasound, surgeons may not be able to locate the residual tumor; therefore, they must depend on the 3D model prepared by the radiologist from the MRI images.
Overall, we can say that the surgical team demonstrated a better understanding of anatomy and lesion localization both preoperatively and intraoperatively. Finally, the 3D virtual model provides a comprehensive view of the sometimes significant and multi-localized metastatic disease. This overarching perspective guides the strategy toward anatomical resections of adjacent lesions and particularly enables systematization in the sequencing of resections. Hepatic surgical management aided by 3D imaging proves to be especially promising. It encourages continuing the project with the aim of providing the best conditions for the success of the surgical procedure to the patient’s benefit. Indeed, surgery assisted by AI is just in its infancy, and we are at the dawn of a technological revolution. The possibilities for development are numerous, and the future is highly promising. Indeed, some leading AI specialists have considered the future of “super surgeons” assisted by AI. Just like in radiology, the prognoses are the same. Likely, surgeons (and radiologists) who do not wish to use AI will quickly become outdated, whereas those who use it as an additional tool will quickly gain an advantage over others. Computers and algorithms will not immediately replace the doctors of tomorrow, but they will have to get used to working with AI to stay at the forefront of medicine, as our predecessors strived to do. The future of surgery also involves robotic surgery. This could be significantly enhanced by holograms and/or 3D modeling[18]. Some surgeons are already advocating for integrating 3D modeling into robotic systems so that they can verify the patient’s anatomy at any time while performing laparoscopy [Figure 6].
Figure 6. Visualization of a virtual 3D Surgiprint model in the operating room. All medical staff can better understand the patient’s anatomy and follow the surgical procedure. 3D: Three dimensions.
In conclusion, 3D models can assist in detailed anatomical analysis, helping to clarify the spatial relationships between the tumor and the intrahepatic vessels of patients. Virtual 3D models displayed on a two-dimensional screen can obscure certain structures from each other, which hinders the spatial understanding of the image, especially in cases of complex anatomy. Medical staff such as radiologists, surgeons, and operating room nurses must be trained to develop the necessary skills for processing medical images like CT or MRI through the segmentation process to create patient-specific 3D models. These models can be used successfully to better understand the anatomy, plan procedures, and create patient-specific 3D models, which, to some extent, will greatly improve surgery for the benefit of the patient.
The application of 3D navigation solutions in liver surgery is just beginning. Specialized research and industrial groups are developing solutions so that the use of 3D models can be used in routine practice.
One of the weaknesses of virtual and physical 3D models is that they lack a tactile sensation for surgeons. However, 3D remains very promising, especially in the field of liver transplantation, and more specifically in the case of living donor liver transplant (LDLT), where 3D printed models of the donor’s liver and models of the recipient’s anatomy can be made and help to further improve outcomes.
Limits of the study
1. Sample Size: Eight patients represent a sample size that is too small to yield statistically significant results.
2. MRI and 3D Reconstruction: There are challenges with slice thickness and image quality. The thickness of the MRI slices used in this study was 5 mm. This large thickness may, therefore, hinder the accuracy of the reconstruction of 3D models. Typically, CT scans with slices smaller than 1 mm are used for 3D reconstructions, allowing for better results. MRI is superior for determining the number and quality of nodules post-chemotherapy (viable or necrotic lesions), but it is not perfect for helping software achieve precise reconstructions.
3. Subgroups: The sample of people taking part in the study is also small (12), which can lead to a reduction in the quality and accuracy of the study.
4. Weakness of results: Concordance between individual localizations was high only for senior radiologists. All readers localized lesions better with basic MRI than with 3D, except for senior surgeons. These results suggest that the benefits of 3D are mainly significant for the most experienced surgeons and radiologists, thus limiting the tool’s utility. The goal of 3D is to better define and locate lesions and to guide HPB surgeons in precise surgical planning.
It is difficult to draw conclusions from analyzing 8 patients divided among 4 subgroups of physicians. It was foreseeable that a project based on 3D reconstruction using MRI would not yield very robust results. In the future, it would be interesting to compare a larger number of patients and to use CT alongside MRI for 3D reconstruction.
Another weakness of the 3D model is the time it takes to create a liver model, which can take up to 10 days, and its cost, which is around $700. However, techniques are developing every day, which helps to reduce both the creation time and, consequently, the production cost.
Future and prospects
The future of virtual 3D modeling for liver metastases holds incredible potential, and we can envision a truly transformative impact on hepatic surgery and cancer treatment. Imagine a world where surgeons and radiologists no longer rely on static, two-dimensional images but instead immerse themselves in fully interactive 3D environments, where every detail of a patient’s liver is reconstructed in ultra-high resolution.
Advanced AI-assisted planning
In the near future, AI will not just assist in creating 3D models but will predict optimal surgical pathways based on tumor growth, blood vessel proximity, and liver health. Surgeons could receive real-time feedback on the safest and most efficient resection strategies, with simulations running multiple scenarios in seconds, helping avoid complications during surgery.
AR and VR integration
Take a step further: augmented reality (AR) and VR will be fully integrated into operating rooms. Surgeons could wear AR glasses to visualize the 3D liver model overlaid on the patient’s body during surgery, guiding them through complex resections with unparalleled precision. They could even manipulate the virtual liver model using gestures, zooming in on metastases, visualizing vascular structures, or rotating the liver to get the best angles while preserving healthy tissue.
3D-printed organs and holographic surgery assistants
Imagine surgeons practicing on 3D-printed liver replicas before the actual procedure, enhancing their understanding of complex cases. These models would not just represent the anatomy but could even mimic the texture and density of the liver, allowing for realistic simulation. Holographic assistants could also join the surgical team, offering on-the-spot advice and real-time anatomical adjustments based on the evolving conditions during surgery.
Remote surgeries and global collaboration
With 3D models accessible anywhere, global collaboration becomes seamless. A surgeon in Europe could consult in real time with an expert in the United States or Asia, projecting the same 3D model into their respective AR systems. Surgeons could even perform remote surgeries, guiding robotic tools across the globe using 3D modeling technology to achieve the highest precision.
Next-generation tumor monitoring
On the diagnostic front, advanced 3D modeling will soon integrate real-time imaging and AI-driven monitoring systems to track the smallest changes in tumor size or activity. Wearable devices could feed continuous patient data into the model, offering a real-time, dynamic understanding of how chemotherapy, immunotherapy, or radiotherapy is affecting metastatic liver lesions.
These advancements promise to revolutionize the way we understand, plan, and perform hepatic surgeries for liver metastases, bringing precision, safety, and personalization to levels we can barely imagine today. The future is not just about better outcomes - it is about completely reimagining the surgeon’s toolkit for unparalleled effectiveness.
DECLARATIONS
Authors’ contributions
Made substantial contributions to the conception and design of the study and performed data analysis and interpretation: Salavracos M, de Hemptinne A, De Poortere T
Performed data acquisition, as well as providing administrative, technical, and material support: Salavracos M, Coubeau L
Availability of data and materials
Data is not available due to that the patients of this study did not agree for their data to be shared publicly.
Financial support and sponsorship
None.
Conflicts of interest
All authors declared that there are no conflicts of interest.
Ethical approval and consent to participate
Informed consent was gathered from each participant, and this study was approved by the CEHF Comité d’Ethique Hospitalo-Facultaire Saint-Luc UCLouvain (2021/27OCT/446. Belgian registration number B403).
Consent for publication
I declare that the consent form has been obtained by all patients.
Copyright
© The Author(s) 2024.
REFERENCES
1. Cronin KA, Lake AJ, Scott S, et al. Annual report to the nation on the status of cancer, part I: national cancer statistics. Cancer 2018;124:2785-2800.
3. Siegel RL, Miller KD, Jemal A. Colorectal cancer mortality rates in adults aged 20 to 54 years in the United States, 1970-2014. JAMA 2017;318:572-4.
4. Steele G Jr, Ravikumar TS. Resection of hepatic metastases from colorectal cancer. Biologic perspective. Ann Surg 1989;210:127-38.
5. Blumgart LH, Allison DJ. Resection and embolization in the management of secondary hepatic tumors. World J Surg 1982;6:32-45.
6. Riihimäki M, Hemminki A, Sundquist J, Hemminki K. Patterns of metastasis in colon and rectal cancer. Sci Rep 2016;6:29765.
7. Lafortune M, Denys A, Sauvanet A, Schmidt S. Anatomy of the liver: what you need to know. J Radiol 2007;88:1020-35.
8. Brandi G, Ricci AD, Rizzo A, et al. Is post-transplant chemotherapy feasible in liver transplantation for colorectal cancer liver metastases? Cancer Commun 2020;40:461-4.
9. Soon DSC, Chae MP, Pilgrim CHC, Rozen WM, Spychal RT, Hunter-Smith DJ. 3D haptic modelling for preoperative planning of hepatic resection: a systematic review. Ann Med Surg 2016;10:1-7.
10. Ballard DH, Wake N, Witowski J, Rybicki FJ, Sheikh A. RSNA Special Interest Group for 3D Printing Abdominal; Hepatobiliary; and Gastrointestinal Conditions Voting Group. Radiological Society of North America (RSNA) 3D Printing Special Interest Group (SIG) clinical situations for which 3D printing is considered an appropriate representation or extension of data contained in a medical imaging examination: abdominal, hepatobiliary, and gastrointestinal conditions. 3D Print Med 2020;6:13.
11. Wang JZ, Xiong NY, Zhao LZ, Hu JT, Kong DC, Yuan JY. Review fantastic medical implications of 3D-printing in liver surgeries, liver regeneration, liver transplantation and drug hepatotoxicity testing: a review. Int J Surg 2018;56:1-6.
12. Moris D, Ronnekleiv-Kelly S, Rahnemai-Azar AA, et al. Parenchymal-sparing versus anatomic liver resection for colorectal liver metastases: a systematic review. J Gastrointest Surg 2017;21:1076-85.
13. FDA. Indications for Use - syngo.CT liver analysis. Available from: https://www.accessdata.fda.gov/cdrh_docs/pdf13/K133643.pdf. [Last accessed on 28 Oct 2024].
14. Igami T, Nakamura Y, Hirose T, et al. Application of a three-dimensional print of a liver in hepatectomy for small tumors invisible by intraoperative ultrasonography: preliminary experience. World J Surg 2014;38:3163-6.
15. Mise Y, Aloia TA, Brudvik KW, Schwarz L, Vauthey JN, Conrad C. Parenchymal-sparing hepatectomy in colorectal liver metastasis improves salvageability and survival. Ann Surg 2016;263:146-52.
16. Fretland ÅA, Dagenborg VJ, Bjørnelv GMW, et al. Laparoscopic versus open resection for colorectal liver metastases: the OSLO-COMET randomized controlled trial. Ann Surg 2018;267:199-207.
17. Barimani D, Kauppila JH, Sturesson C, Sparrelid E. Imaging in disappearing colorectal liver metastases and their accuracy: a systematic review. World J Surg Oncol 2020;18:264.
Cite This Article
How to Cite
Salavracos, M.; de Hemptinne, A.; De Poortere, T.; Coubeau, L. Contribution of 3D virtual modeling in locating hepatic metastases, particularly “vanishing tumors”: a pilot study. Art. Int. Surg. 2024, 4, 331-47. http://dx.doi.org/10.20517/ais.2024.23
Download Citation
Export Citation File:
Type of Import
Tips on Downloading Citation
Citation Manager File Format
Type of Import
Direct Import: When the Direct Import option is selected (the default state), a dialogue box will give you the option to Save or Open the downloaded citation data. Choosing Open will either launch your citation manager or give you a choice of applications with which to use the metadata. The Save option saves the file locally for later use.
Indirect Import: When the Indirect Import option is selected, the metadata is displayed and may be copied and pasted as needed.
About This Article
Copyright
Data & Comments
Data
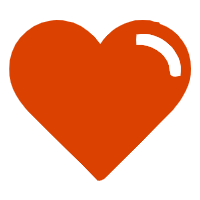
Comments
Comments must be written in English. Spam, offensive content, impersonation, and private information will not be permitted. If any comment is reported and identified as inappropriate content by OAE staff, the comment will be removed without notice. If you have any queries or need any help, please contact us at support@oaepublish.com.