Non-atherosclerotic coronary artery disease in molecular autopsy: a literature review
Abstract
Non-atherosclerotic coronary artery disease (N-ACAD) is a group of conditions affecting the coronary arteries that can result in sudden cardiac death. This group of conditions can be categorized into structural anomalies, such as collagenopathies, and functional anomalies, such as inflammatory vasculopathies, based on the pathological mechanism. The epidemiology of N-ACAD varies according to the genetic condition involved; however, these disorders are thought to represent a significant proportion of sudden cardiac deaths among young individuals, especially those with a family history of inherited cardiovascular diseases. In collagenopathies, the causes of
Keywords
INTRODUCTION
Cardiovascular diseases are the leading cause of death worldwide[1], with coronary artery disease (CAD) being the most significant cause of morbidity and mortality[2,3]. CAD encompasses a group of diseases affecting the coronary arteries and can be categorized into atherosclerotic CAD (ACAD) and
SCDY is a major international public health issue and is defined as SCD affecting individuals aged under
In contrast to CAD, which is largely linked to chronic inflammation and environmental influences,
CLASSIFICATION
N-ACAD can be divided into collagenopathies, characterized by alterations of the connective tissue, and vasculitis, characterized by inflammatory alterations [Table 1].
Classification of non-atherosclerotic coronary artery disease
Non-atherosclerotic coronary artery disease | Pathogenic variants | Pathogenetic mechanism | Cardiac complications |
Collagenopathies | |||
Marfan syndrome | FBN1 (90%) TGFBR1 TGFBR2 | Altered formation of connective tissue with consequent weakness of the arterial wall | Dilation, aneurysms or spontaneous coronary dissection and sudden cardiac death in the young |
Vascular Ehlers-Danlos syndrome | COL3A1 | Defect in collagen production resulting in vessel fragility | Spontaneous coronary dissections, arterial ruptures and coronary aneurysms, and sudden cardiac death in the young |
Loeys-Dietz syndrome | TGFBR1 TGFBR2 SMAD3 TGFB2 TGFB3 SMAD2 | Dysregulation of TGF-β signaling resulting in vascular wall weakness | Aneurysm, spontaneous coronary dissection, sudden cardiac death in the young and abnormal tortuosity |
Vasculitis | |||
Kawasaki disease | ITPKC, CASP3, FCGR2A, BLK, CD40, NAALADL2, ZFHX3, PELI1, COPB2, ERAP1, S100A12, MMP-9, TLR2, NLRC4, ARG1 | Systemic vasculitis of medium and small vessels of unknown etiopathogenesis due to probable immune system dysfunction | Coronary aneurysms, ischemia and sudden cardiac death in the young |
Takayasu arteritis | IL12B, MICA-1.2, LILRB3, LILRA3, IL6, FCGR2A/FCGR3A | Chronic idiopathic granulomatous vasculitis of the large vessels of unknown etiopathogenesis due to probable immune system dysfunction | Coronary artery stenosis or occlusion, diffuse or focal coronary arteritis, coronary artery aneurysm, resulting in myocardial ischemia and arrhythmias, and sudden cardiac death in the young |
Collagenopathies include syndromic conditions such as Marfan syndrome (MFS), vascular Ehlers-Danlos syndrome (VEDS), and Loeys-Dietz syndrome (LDS), which cause alterations in the walls of the coronary arteries, predisposing individuals to spontaneous coronary artery dissection (SCAD) or coronary aneurysms, as well as sudden cardiac death in the young SCDY.
Vasculitis includes inflammatory CAD, such as Takayasu arteritis (TA) and Kawasaki disease (KD), which are primarily mediated by an abnormal immune response and associated with multifactorial pathogenesis involving genetic, environmental, and infectious factors. In these diseases, immune system dysfunction leads to an inflammatory response in the blood vessels. Autoantibodies and T cells involved in the immune response contribute to vascular inflammation and tissue injury, affecting the coronary arteries and posing a potential risk of ischemia or aneurysms, as well as SCDY.
COLLAGENOPATHIES IN SUDDEN CARDIAC DEATH IN THE YOUNG
Marfan syndrome
MFS is an inherited disorder of connective tissue, with approximately 90% of cases attributed to pathogenic variants in the FBN1 gene[10], and less frequently to mutations in either TGFBR1 or TGFBR2 genes[11]. These genetic alterations are linked to the development of aneurysms and arterial dissections, predominantly affecting the aorta but also involving the coronary arteries, and they represent a major cause of sudden cardiac death among young individuals[12] [Figure 1].
Figure 1. MFS genetic mechanisms. MFS: Marfan syndrome; SCD: sudden cardiac death; SCAD: spontaneous coronary artery dissection.
MFS is the most prevalent form of collagenopathy, occurring in approximately 1 in every 5,000 individuals[13]. It affects people of all sexes and ethnic backgrounds equally[11]. Without appropriate treatment, the condition is associated with a significantly reduced life expectancy, averaging around 40 years[14].
MFS follows an autosomal dominant inheritance pattern with complete penetrance and variable expressivity. Approximately 25% of cases are sporadic, resulting from de novo mutations[15]. The FBN1 gene, located on chromosome 15q21.1, encodes fibrillin-1, a glycoprotein that is a key component of the extracellular matrix in elastic fibers. The majority of FBN1 mutations are single nucleotide changes, with missense variants accounting for approximately 60% and nonsense mutations representing around 10%. In fewer than 10% of cases, the mutation may go undetected, often due to complete deletion of the allele or disruptions in gene regulation[10].
The TGFBR1 and TGFBR2 genes encode the transforming growth factor-beta receptor (TGFBR), which may be mutated in atypical presentations of MFS[11]. Fibrillin-1 regulates the bioavailability of TGF-β, a growth factor involved in processes such as inflammation and tissue repair. Mutations in fibrillin-1 lead to overexpression of TGF-β, making it more active and available. This overactivity promotes inflammation, tissue fibrosis, and the activation of matrix metalloproteinases (MMPs), particularly MMP-2 and MMP-9[16].
These enzymes degrade structural components of the extracellular matrix, such as collagen and elastin, weakening arterial walls and resulting in dilation, aneurysms, or SCAD[17,18], which can culminate in sudden cardiac death.
Histologically [Table 2], MFS is marked by regions of cystic medial degeneration, disruption of elastic fibers across the lamellae, depletion of smooth muscle cells, and fragmentation of the elastic tissue within the tunica media[19].
Histology and causes of death in collagenopathies
Collagenopathies | Histology | Causes death |
Marfan syndrome | Cystic degeneration of the tunica media, fragmentation of elastic fibers, loss of smooth muscle cells | Aortic and coronary dissections |
Vascular Ehlers-Danlos syndrome | Cystic degeneration of the tunica media, fragmentation of elastic fibers | Arterial ruptures and coronary dissections |
Loeys-Dietz syndrome | Cystic degeneration of the media, intralamellar elastic fragmentation, loss of smooth muscle cells | Dissections and aneurysms |
Vascular Ehlers-Danlos syndrome
VEDS is a hereditary connective tissue disorder caused by pathogenic variants in the COL3A1 gene. It is characterized by extreme fragility of blood vessels[20] [Figure 2].
Figure 2. VEDS genetic mechanisms. SCD: Sudden cardiac death; SCAD: spontaneous coronary artery dissection; VEDS: vascular Ehlers-Danlos syndrome.
VEDS represents the most severe subtype of Ehlers-Danlos syndrome (EDS) and is characterized by a significantly elevated risk of cardiovascular complications. While it primarily affects the aorta, it also predisposes individuals to the rupture of small-to-medium caliber vessels, including the coronary arteries, leading to spontaneous coronary dissections[21], arterial ruptures, and coronary aneurysms, which can result in sudden cardiac death, particularly in young individuals[22].
VEDS is an exceedingly rare condition, representing only 5% of all EDS cases[23], with a prevalence of approximately 1 in 150,000 individuals[24]. It affects both genders equally[23], although sudden death before the age of 20 is more frequently observed in males[25]. VEDS follows an autosomal dominant inheritance pattern, exhibiting high penetrance[20].
The COL3A1 gene, situated on chromosome 2q32.2, encodes type III collagen, a key structural protein essential for the stability of connective tissues, particularly within blood vessels[26]. In half of the cases, pathogenic variants in COL3A1 involve missense mutations that substitute glycine in the Gly-X-Y repeat sequence of the collagen triple helix. Less commonly, pathogenic variants result in haploinsufficiency, which is associated with less severe phenotypic expressions, while bi-allelic mutations occur in fewer than 1% of cases. Furthermore, heterozygous substitutions of arginine to cysteine in COL1A1 can occasionally produce a clinical presentation resembling VEDS[26].
Histologically [Table 2], VEDS is characterized by areas of cystic degeneration in the tunica media and translamellar medial elastin fragmentation[19].
Loeys-Dietz syndrome
LDS is a disorder of connective tissue caused by pathogenic mutations in genes that code for TGF-β receptors and other related proteins within the same signaling pathway. These genetic alterations impair TGF-β signaling, disrupting the normal formation and upkeep of connective tissue, which leads to weakened arterial walls and vascular abnormalities[27] [Figure 3].
Figure 3. LDS genetic mechanisms. LDS:Loeys-Dietz syndrome; SCD: sudden cardiac death; SCAD: spontaneous coronary artery dissection.
Vascular anomalies in LDS can occasionally involve the coronary arteries[28], manifesting as aneurysms, spontaneous coronary dissections[29], and in severe cases, sudden cardiac death in young individuals[27]. Abnormal arterial tortuosity is also a common feature[30]. LDS is particularly dangerous in young people, with dissections often occurring suddenly during childhood or adolescence.
LDS is a rare disorder[31], with its incidence and prevalence not yet fully established. It affects both males and females across diverse ethnic groups, with cardiovascular complications being the primary cause of morbidity and mortality among younger individuals[32]. LDS follows an autosomal dominant inheritance pattern[33], with around two-thirds of cases arising from de novo mutations, which are typically linked to more severe phenotypes. The remaining cases are familial, often presenting with less pronounced symptoms. There have also been reports of non-penetrance and mosaicism in certain cases[34].
The primary pathogenic variants associated with LDS include TGFBR1 and TGFBR2, which encode type 1 and type 2 TGF-β receptors; SMAD3, which encodes a protein mediating intracellular TGF-β signaling; and TGFB2 and TGFB3, which encode TGF-β isoforms 2 and 3[34-36]. LDS is classified into five primary subtypes, each linked to a specific genetic mutation. The first subtype, LDS Type 1, is associated with mutations in the TGFBR1 gene, while LDS Type 2 results from mutations in the TGFBR2 gene. Another subtype, LDS Type 3, is caused by changes in the SMAD3 gene. LDS Type 4 is linked to mutations in the TGFB2 gene, and finally, LDS Type 5 arises from mutations in the TGFB3 gene[36]. Pathogenic variants in the SMAD2 gene have also been discovered, although these have not yet been assigned to a specific subtype of LDS[37].
Histologically [Table 2], LDS is characterized by regions of cystic degeneration within the tunica media, along with extensive intralamellar fragmentation of medial elastin. There is also a loss of smooth muscle cells and disruption of elastic fibers, including their fragmentation and disorganization[19].
VASCULITIS IN SUDDEN CARDIAC DEATH IN THE YOUNG
Kawasaki disease
KD, also known as mucocutaneous lymph node syndrome, is an acute, self-limiting systemic vasculitis that affects medium and small vessels, with a particular affinity for the coronary arteries[38].
The exact cause of KD remains unknown, though it is believed to have a multifactorial origin, and includes genetic predisposition, environmental factors, and infections [Figure 4]. Various viral and bacterial pathogens, such as Epstein-Barr virus (EBV), adenovirus, and bacterial superantigens such as streptococci and staphylococci, have been implicated as possible triggers of the disease. The involvement of the immune system is crucial, with an inflammatory response characterized by the activation of T lymphocytes, macrophages and the release of proinflammatory cytokines, leading to endothelial damage and thrombosis[39].
KD is the most common vasculitis in newborns and children under 5 years of age, predominantly affecting males and those of Asian descent. It is more prevalent during the winter and spring months. The incidence ranges from 10-20 per 100,000 children under age 5 in the United States and Canada to 50-250 per 100,000 in Japan, Taiwan, and Korea[40].
KD predominantly involves the coronary arteries, with a reported frequency of 25%-30%[41]. Among affected coronary arteries, the right coronary artery is most commonly involved (35%), followed by the anterior descending coronary artery (32%)[42]. KD is the leading cause of coronary artery aneurysms in children, which can lead to sudden cardiac death[38,43].
The role of the HLA region in KD has been studied with mixed results[44]. While some HLA alleles, such as HLA-Bw51, have shown associations with KD in various populations, these findings are not always consistent. A study on Japanese patients identified strong signals involving HLA-DQB2 and HLA-DOB[45], though no specific HLA allele universally associated with KD susceptibility has been confirmed[46].
Genomic studies have identified additional genes linked to KD, including ITPKC, CASP3, FCGR2A, BLK, and CD40, which are involved in immune and inflammatory processes. Other implicated genes include NAALADL2, ZFHX3, PELI1, COPB2, and ERAP1. More recently, key “hub” genes such as S100A12, MMP-9, TLR2, NLRC4, and ARG1 have been recognized for their critical roles in the immune processes underlying KD[44-46]. Genetic studies have also linked specific variants to an increased risk of coronary artery aneurysms and thrombosis in children with KD[44,47,48].
Histological analysis of KD [Table 3] reveals a polymorphic inflammatory infiltrate within the thinned walls of blood vessels. This process is accompanied by a progressive loss and destruction of elastic fibers, ultimately leading to necrosis of the internal elastic lamina[38,42].
Histology and causes of death in vasculitis
Vasculitis | Stage-phase disease | Histology | Causes death |
Kawasaki disease | Polymorphous inflammatory infiltrate in thinned vascular walls; rarefaction and destruction of elastic fibers; necrosis of the internal elastic lamina | ||
Stage I (0-9 days) | Perivasculitis and acute vasculitis of microvessels (arterioles, capillaries, venules) and small arteries; perivasculitis and acute endarteritis of the three main coronary arteries; pericarditis, myocarditis, inflammation of the atrioventricular conduction system, endocarditis with valvulitis | Heart failure or arrhythmias | |
Stage II (12-25 days) | Panvasculitis of the three main coronary arteries; aneurysm formation with thrombi; myocarditis; coagulative necrosis; conduction system lesions; persistent pericarditis and valvulitis | Myocardial infarction, ruptured aneurysms, heart failure or arrhythmias | |
Stage III (28-31 days) | Granulation tissue in the main coronary arteries; resolution of microvascular inflammation | Myocardial infarction | |
Stage IV (40 days-4 years) | Scarring and severe stenosis of the main coronary arteries; myocardial fibrosis; coagulative necrosis; conduction system lesions; endocardial fibroelastosis | Myocardial infarction | |
Takayasu arteritis | Intimal hyperplasia and granulomatous arteritis. Stenosis or occlusion of the coronary arteries; diffuse or focal coronary arteritis; coronary aneurysms | Myocardial ischemia and arrhythmias | |
Acute phase | Edema; patchy necrosis; chronic inflammation; scattered giant cells, primarily in the outer two-thirds of the tunica media, adventitia, adventitial fat, and vasa vasorum. Intimal proliferation with obliteration of the lumen | ||
Chronic phase | Marked intimal and adventitial thickening, leading to multi-segmental circumferential stenosis. Hypocellular intimal proliferation with scattered fibroblasts and smooth muscle cells. Disorganized or absent elastic fibers, replaced by collagen and granulation tissue. Presence of necrosis with giant infiltrates |
Cardiac lesions in KD develop over time and are classified into different stages based on the duration of the disease at the time of death[49,50].
In Stage I from 0 to 9 days, the earliest phase, inflammation primarily affects the microvessels such as arterioles, capillaries, and venules and small arteries, leading to perivasculitis and acute vasculitis. The three main coronary arteries also show signs of inflammation, with perivasculitis and acute endarteritis. Other cardiac complications, such as pericarditis, myocarditis, and inflammation of the atrioventricular conduction system, may also occur. Endocarditis and valvulitis further contribute to the damage. In this stage, death is most commonly caused by heart failure or fatal arrhythmias.
During Stage II from 12 to 25 days, the inflammation progresses to involve all layers of the three major coronary arteries, leading to widespread pan-vasculitis. This stage is also marked by the formation of aneurysms, often containing thrombi within the main arterial stems. Myocarditis and coagulative necrosis persist, along with ongoing lesions in the conduction system. Pericarditis and valvulitis remain present. At this stage, death can result from complications seen in Stage I, but also from myocardial infarction or the rupture of an aneurysm.
By Stage III from 28 to 31 days, the acute inflammatory response begins to subside, with granulation tissue forming within the three main coronary arteries. At the same time, microvascular inflammation gradually resolves. However, myocardial infarction remains a major risk and is the leading cause of death at this stage.
In Stage IV from 40 days to 4 years, the long-term consequences of the disease become evident. Extensive scarring and severe narrowing of the three major coronary arteries develop, accompanied by myocardial fibrosis and coagulative necrosis. The conduction system remains affected, and endocardial fibroelastosis can also occur. At this advanced stage, myocardial infarction continues to be the most common cause of death.
Takayasu arteritis
TA, also known as Takayasu disease, is a rare, chronic, idiopathic granulomatous vasculitis primarily affecting large vessels, particularly the aortic arch and its branches, including the coronary arteries[51,52].
The exact cause of TA remains unknown, but it is believed that a dysfunctional immune response, mediated by T lymphocytes and macrophages, leads to chronic and progressive inflammation of the vascular walls, resulting in stenosis, thrombosis, and ischemia of the blood supply organs [Figure 5]. Mycobacterium tuberculosis infection has been suggested as a possible trigger, as it can induce abnormal immune activation in genetically predisposed people. Other factors, such as cocaine use and certain bacterial infections, have also been associated with vasculitis cases with similar characteristics[53].
TA predominantly affects young women aged 20-40 years[44], with a higher prevalence in Asian populations[54]. In Japan, the prevalence is approximately 40 per million[55]. Coronary artery involvement occurs in 10%-45% of cases, often targeting the coronary ostia[41,42].
The three most common coronary lesions in TA are:
• Stenosis or occlusion of the coronary arteries;
• Diffuse or focal coronary arteritis;
• Coronary artery aneurysms.
These lesions can result in myocardial ischemia, arrhythmias, and sudden cardiac death.
TA is strongly associated with HLA class I alleles[44], particularly HLA-B52, with a pronounced link in Asian and Mexican populations[56]. Genome-wide association studies (GWAS) have confirmed the relevance of HLA-B5201 and the IL12B gene, especially in Japanese individuals[57].
Other genetic variants have also been implicated, including the MICA-1.2 allele and polymorphisms in the C4 gene[44]. Additional studies have identified two susceptibility loci in Japanese patients, while in other populations, genes such as LILRB3, LILRA3, and IL6, involved in immune regulation and inflammation, have been associated with the disease[58]. Furthermore, a shared association with the FCGR2A/FCGR3A locus has been observed in both TA and Kawasaki disease[44].
Histological analysis [Table 3] shows that TA is characterized by intimal hyperplasia and granulomatous inflammation affecting the arterial walls [38,43].
In the acute phase, the affected arteries exhibit edema, patchy necrosis, and chronic inflammation, often accompanied by scattered giant cells. These changes primarily involve the outer two-thirds of the tunica media, the adventitia, the surrounding fat, and the vasa vasorum. In some cases, the intima also undergoes proliferation, which can result in luminal narrowing or even complete occlusion.
As the disease progresses into the chronic phase, there is significant thickening of both the intima and adventitia, leading to a characteristic multi-segmented, circumferential stenosis. The intima becomes hypocellular, containing only scattered smooth muscle cells and fibroblasts. The normal structure of the arterial wall is disrupted, with elastic fibers becoming disorganized or completely lost, replaced by collagen and granulation tissue. Areas of necrosis persist, often surrounded by infiltrates of giant cells, further contributing to vascular damage and dysfunction.
MOLECULAR AUTOPSY AND GENETIC COUNSELING
SCD is one of the leading causes of mortality worldwide. In young individuals, it is often linked to undiagnosed genetic heart diseases[4]. In such cases, MA - a post-mortem genetic analysis aimed at identifying pathogenic variants associated with hereditary cardiac conditions[59] - is essential [Table 4].
Diagnostic algorithm
Diagnostic phase | Description |
1. SCD assessment | - Sudden death in young people, without apparent cause - Family history of SCD, dissections, aneurysms - Standard autopsy to exclude common pathologies |
2. When to consider MA | - Suspicion of a genetic cardiac disease not detected by standard autopsy - Presence of genetic factors predisposing to channelopathies, cardiomyopathies, aortopathies and coronary artery disease |
3. MA | - Genetic testing for pathogenic variants in genes associated with inherited heart disease: 1. Channelopathies (SCN5A, KCNQ1) 2. Cardiomyopathies (MYH7, LMNA) 3. Aortopathies and connective tissue disorders (FBN1, COL3A1, TGFBR1, TGFBR2) 4. Screen for variants in vasculitis-related genes such as KD, TA, and other genetic abnormalities rarely associated with SCD |
4. Interpretation of molecular results | - Identify pathogenic variants associated with SCD - Confirm the genetic cause of death or exclude rare genetic diseases |
5. Genetic counseling | - Collection of family history (family tree) - Discussion of genetic results and risk of transmission - Psychological support for families |
6. Family clinical surveillance | - Planning of genetic testing for family members - Regular monitoring with echocardiograms, cardiac MRI, stress tests - Preventive surgery: aortic replacement and aneurysm repair |
7. Preventive measures | - Drugs: beta-blockers and angiotensin receptor antagonists - Prophylactic surgery in case of high risk for dissections and aneurysms |
MA plays a crucial role in N-ACAD, where the structural or functional abnormalities responsible for SCD cannot be detected using standard autopsy techniques.
By identifying pathogenic variants linked to conditions such as channelopathies, cardiomyopathies, aortopathies, and CAD, MA can help establish a genetic cause of SCD, particularly in asymptomatic young individuals for whom the first clinical manifestation is a fatal event.
Connective tissue disorders such as MS, VEDS, and LDS are well known to increase the risk of SCAD and aneurysms, both of which can lead to SCDY. These disorders arise from pathogenic variants that impair connective tissue formation, weakening arterial walls.
MS is a hereditary connective tissue disorder primarily caused by pathogenic variants in the FBN1 gene and, less frequently, in TGFBR1 or TGFBR2. These mutations lead to aneurysms and dissections, mainly in the aorta but also in the coronary arteries, significantly increasing the risk of SCDY.
VEDS results from pathogenic variants in the COL3A1 gene, which encodes type III collagen, a protein essential for vascular integrity. It is characterized by extreme vascular fragility, with a high risk of spontaneous coronary dissections, arterial ruptures, and coronary aneurysms, all of which frequently lead to SCDY.
LDS arises from pathogenic mutations in genes that code for TGF-β receptors or associated proteins, leading to impaired TGF-β signaling and resulting in compromised structural integrity of the arterial walls. Vascular abnormalities in LDS include coronary aneurysms, spontaneous coronary dissections, and arterial tortuosity, all of which contribute to the risk of SCDY. Vasculitides such as KD and TA can also contribute to N-ACAD, increasing the risk of SCDY.
KD is associated with genetic variants such as ITPKC and CASP3, as well as genes involved in immune and inflammatory pathways, including FCGR2A, BLK, CD40, NAALADL2, ZFHX3, PELI1, COPB2, ERAP1, S100A12, MMP-9, TLR2, NLRC4, and ARG1. Coronary aneurysms in KD can lead to thrombosis or rupture, potentially causing sudden death even years after the acute phase of the disease.
TA is linked to HLA alleles, particularly HLA-B52. Genetic studies have also identified variants in IL12B, MICA-1.2, and immune-response-related genes such as LILRB3, LILRA3, and IL6. TA can cause coronary stenosis, myocardial ischemia, and myocardial infarction (MI), all of which may ultimately result in SCDY.
Although these conditions are often diagnosed during life, MA plays a vital role in cases of sudden unexplained death, helping to confirm genetic variants that predispose individuals to exaggerated immune responses or impaired vascular repair[60,61]. Beyond providing a definitive diagnosis for the families of the deceased, MA enables genetic screening of relatives, allowing for preventive measures such as clinical monitoring, antiarrhythmic medications, or surgical interventions to mitigate the risks of dissection or aneurysm.
Genetic counseling is essential for managing inherited diseases, particularly those associated with SCD risk. It helps identify, educate, and support families who may be at risk by integrating medical, genetic, and psychological expertise to address the implications of pathogenic variants.
The first step in genetic counseling is gathering a detailed family and personal medical history, often visualized through a family tree that highlights cases of sudden death, vascular dissections, or aneurysms. This is followed by the interpretation of genetic test results, offering clear explanations about the significance of these mutations, their inheritance patterns, and their potential health implications.
Management strategies developed through genetic counseling are multidisciplinary. Preventive approaches include personalized clinical surveillance plans, such as regular echocardiograms, cardiovascular MRI scans, and stress tests to monitor vascular changes. In cases of high risk, prophylactic surgical interventions may be recommended, such as replacing a dilated aorta in patients with MS or repairing coronary aneurysms. Additionally, medications such as beta-blockers or angiotensin receptor antagonists may be prescribed to reduce the risk of dissection or aneurysm in individuals with specific conditions.
From an emotional perspective, genetic counseling provides significant support to patients and their families. Learning that they carry a pathogenic mutation can cause anxiety, guilt, or uncertainty, particularly in families with a history of SCDY. It is, therefore, crucial to emphasize that genetic knowledge is not just a means of identifying risks but, more importantly, a powerful tool for prevention.
Genetic screening for N-ACAD is a topic that, despite being of great interest, does not represent a universal diagnostic solution for all cases of this condition. Although genetics can contribute to the understanding of some forms of N-ACAD, its application is limited to certain patient subgroups for which specific genetic markers have been identified. However, in non-genetic forms of N-ACAD, the diagnosis requires a broader approach, which also includes histological and immunological investigations. These diagnostic tools allow for the analysis of the cellular and molecular mechanisms underlying non-atherosclerotic coronary heart disease, providing a more precise and comprehensive view compared to genetic tests, which are not always able to detect all the pathogenetic factors involved. Histological analyses, in fact, allow for the examination of tissues and the identification of morphological and inflammatory characteristics that may underline
FUTURE DIRECTIONS AND CHALLENGES
AM represents a promising frontier in precision medicine, enabling the integration of histopathological data with detailed genomic analyses. The use of emerging technologies such as high-throughput sequencing and single-cell sequencing offers new opportunities to refine the diagnosis of N-ACAD, enhancing early detection and risk assessment. In particular, single-cell sequencing allows for the analysis of genetic material expressed in thousands of individual cells, revealing significant differences between them and providing a deeper understanding of the pathological microenvironment[61].
On the therapeutic front, gene editing emerges as a transformative strategy to correct pathogenic mutations underlying N-ACAD. Techniques such as CRISPR/Cas9 enable precise DNA interventions, paving the way for personalized treatments for a wide range of genetic diseases[62]. In parallel, the development of targeted therapies based on an understanding of the specific molecular mechanisms of N-ACAD could enhance treatment efficacy and reduce side effects.
However, the implementation of these technologies presents significant challenges. It is essential to validate biomarkers identified through large-scale studies and ensure the safety and effectiveness of new therapies. Furthermore, the integration of big data and artificial intelligence could further enhance diagnostic and prognostic capabilities but requires the development of adequate infrastructure and the resolution of ethical and privacy concerns. Addressing these challenges will be crucial in translating scientific discoveries into concrete clinical applications for the management of N-ACAD.
CONCLUSION
N-ACAD caused by pathogenic variants presents a significant clinical challenge, particularly as a major contributor to sudden cardiac death in seemingly healthy young individuals.
The advent of MA has revolutionized post-mortem diagnosis, not only providing vital answers to the families of the deceased but also serving as a crucial tool for preventing fatal events in genetically predisposed relatives.
Early identification of genetic mutations, followed by the implementation of targeted preventive strategies for at-risk family members, can significantly reduce the incidence of fatal cardiac events.
However, genetic screening for N-ACAD is not a universal diagnostic solution. While it plays a key role in identifying certain genetic forms of the condition, non-genetic cases require a broader diagnostic approach, incorporating histological and immunological investigations. These methods offer deeper insights into the underlying disease mechanisms and are essential when genetic testing alone is inconclusive.
A comprehensive approach that integrates genetic, histological, and immunological techniques is essential for improving both the diagnosis and management of N-ACAD. Consequently, a multidisciplinary strategy is indispensable for the effective prevention of SCD.
DECLARATIONS
Authors’ contributions
Conceptualization: Salzillo C, Marzullo A
Methodology: Salzillo C, Lucà S, Cazzato G
Resources: Salzillo C, Lucà S, Ronchi A
Writing-original draft preparation: Salzillo C, Lucà S, Cazzato G
Writing-review and editing: Salzillo C, Ronchi A, Marzullo A
Supervision: Marzullo A, Ingravallo G
All authors have read and agreed to the published version of the manuscript.
Availability of data and materials
Not applicable.
Financial support and sponsorship
None.
Conflicts of interest
All authors declared that there are no conflicts of interest.
Ethical approval and consent to participate
Not applicable.
Consent for publication
Not applicable.
Copyright
© The Author(s) 2025.
REFERENCES
1. Spînu M, Onea LH, Homorodean C, Olinic M, Ober MC, Olinic DM. Optical coherence tomography-OCT for characterization of non-atherosclerotic coronary lesions in acute coronary syndromes. J Clin Med. 2022;11:265.
2. Herrington W, Lacey B, Sherliker P, Armitage J, Lewington S. Epidemiology of atherosclerosis and the potential to reduce the global burden of atherothrombotic disease. Circ Res. 2016;118:535-46.
3. D'Abbronzo G, Franco R, Salzillo C, et al. Application of digital analysis for assessment of coronary sub-occlusions in autopsy pathology: it is time to move beyond histology alone. Diagnostics. 2024;14:2115.
4. Salzillo C, Sansone V, Napolitano F. Sudden cardiac death in the young: state-of-the-art review in molecular autopsy. Curr Issues Mol Biol. 2024;46:3313-27.
6. Corrado D, Basso C, Thiene G. Sudden cardiac death in young people with apparently normal heart. Cardiovasc Res. 2001;50:399-408.
7. Risgaard B. Sudden cardiac death: a nationwide cohort study among the young. Dan Med J. ;63:B5321.
8. Tomassini L, Ricchezze G, Fedeli P, et al. New insights on molecular autopsy in sudden death: a systematic review. Diagnostics. 2024;14:1151.
9. Skrypnyk C, AlHarmi R. Molecular autopsy by proxy: relevance for genetic counseling in rare genetic disorders. Front Genet. 2024;15:1400295.
10. Monda E, Caiazza M, Limongelli G. The role of genetic testing in Marfan syndrome. Curr Opin Cardiol. 2024;39:162-9.
11. Salik I, Rawla P. Marfan syndrome. In: StatPearls [Internet]. Treasure Island (FL): StatPearls Publishing; 2025.
12. Mitrofanova LB, Rybakova MG. Causes and mechanisms of sudden cardiac death in children. Sud Med Ekspert. 2021;64:43-9.
13. Haruyama S, Torishima M, Kawasaki H, Wada T, Kosugi S. Decision-making processes behind seeking regular cardiac checkups for individuals with Marfan syndrome: a grounded theory study. J Genet Couns. 2024;33:1191-203.
14. Vanem TT, Geiran OR, Krohg-Sørensen K, Røe C, Paus B, Rand-Hendriksen S. Survival, causes of death, and cardiovascular events in patients with Marfan syndrome. Mol Genet Genomic Med. 2018;6:1114-23.
15. Marelli S, Micaglio E, Taurino J, et al. Marfan syndrome: enhanced diagnostic tools and follow-up management strategies. Diagnostics. 2023;13:2284.
16. Zeigler SM, Sloan B, Jones JA. Pathophysiology and pathogenesis of Marfan syndrome. In: Halper J, editor. Progress in heritable soft connective tissue diseases. Cham: Springer International Publishing; 2021. pp. 185-206.
17. Blech B, Dhamija R, Ingall T. Marfan syndrome presenting as spontaneous coronary artery dissection and arteriopathy. Neurologist. 2021;27:34-6.
18. Murad AM, Hill HL, Wang Y, et al. GenTAC Investigators; Montalcino Aortic Consortium Investigators. Spontaneous coronary artery dissection is infrequent in individuals with heritable thoracic aortic disease despite partially shared genetic susceptibility. Am J Med Genet A. 2022;188:1448-56.
19. Halushka MK, Angelini A, Bartoloni G, et al. Consensus statement on surgical pathology of the aorta from the society for cardiovascular pathology and the association for European cardiovascular pathology: II. noninflammatory degenerative diseases - nomenclature and diagnostic criteria. Cardiovasc Pathol. 2016;25:247-57.
20. Assavarittirong C, Au TY, Nguyen PV, Mostowska A. Vascular Ehlers-Danlos syndrome: pathological variants, recent discoveries, and theoretical approaches. Cardiol Rev. 2022;30:308-13.
21. Bertin L, Hauguel-Moreau M, Prati G, et al. Spontaneous coronary artery rupture in patients with Ehlers-Danlos syndrome: mini review. Cardiovasc Revasc Med. 2022;40:231-4.
22. Buso G, Corvini F, Fusco EM, et al. Current evidence and future perspectives in the medical management of vascular Ehlers-Danlos syndrome: focus on vascular prevention. J Clin Med. 2024;13:4255.
23. Alqahtani M, Claudinot A, Gaudry M, et al. Endovascular management of vascular complications in Ehlers-Danlos syndrome type IV. J Clin Med. 2022;11:6344.
24. Frank M, Albuisson J, Ranque B, et al. The type of variants at the COL3A1 gene associates with the phenotype and severity of vascular Ehlers-Danlos syndrome. Eur J Hum Genet. 2015;23:1657-64.
25. Byers PH, Belmont J, Black J, et al. Diagnosis, natural history, and management in vascular Ehlers-Danlos syndrome. Am J Med Genet C Semin Med Genet. 2017;175:40-7.
26. Demirdas S, van den Bersselaar LM, Lechner R, et al. Vascular Ehlers-Danlos syndrome: a comprehensive natural history study in a dutch national cohort of 142 patients. Circ Genom Precis Med. 2024;17:e003978.
27. Jost ZT, Sang CJ 3rd, Lertwilaiwittaya P, Chapman GD. Coronary artery aneurysm in Loeys-Dietz syndrome: a case report. Eur Heart J Case Rep. 2022;6:ytac204.
28. Nickol JL, Malik SA, Yetman AT. Case report of Loeys-Dietz syndrome presenting with coronary artery aneurysm. Eur Heart J Case Rep. 2022;6:ytac383.
29. Verstraeten A, Perik MHAM, Baranowska AA, et al. European/International Fibromuscular dysplasia registry and initiative (FEIRI); collaborators of the european/international fibromuscular dysplasia registry and initiative (FEIRI). Enrichment of rare variants in Loeys-Dietz syndrome genes in spontaneous coronary artery dissection but not in severe fibromuscular dysplasia. Circulation. 2020;142:1021-4.
30. Chmielewski P, Ponińska JK, Michalak E, et al. Cardiovascular involvement and prognosis in Loeys-Dietz syndrome. Kardiol Pol. 2023;81:1096-102.
31. Gouda P, Kay R, Habib M, Aziz A, Aziza E, Welsh R. Clinical features and complications of Loeys-Dietz syndrome: a systematic review. Int J Cardiol. 2022;362:158-67.
32. Dey S, Cheikhali R, Frishman WH, Aronow WS. Genetic problems, diagnosis, and cardiovascular manifestations of Loeys-Dietz syndrome. Cardiol Rev. 2024;32:513-8.
33. Tian M, Si MS, Zhang Y, Ding Y, Chen J. Surgical treatment of Loeys-Dietz syndrome in a 3-year-old: case report and review of literature. Transl Pediatr. 2020;9:695-701.
34. Velchev JD, Van Laer L, Luyckx I, Dietz H, Loeys B. Loeys-Dietz syndrome. In: Halper J, editor. Progress in heritable soft connective tissue diseases. Cham: Springer International Publishing; 2021. pp. 251-64.
35. Loeys BL, Dietz HC. Loeys-Dietz syndrome. In: Adam MP, Feldman J, Mirzaa GM, et al., editors. GeneReviews® [Internet]. Seattle (WA): University of Washington, Seattle; 2024.
36. Van Laer L, Dietz H, Loeys B. Loeys-Dietz syndrome. In: Halper J, editor. Progress in heritable soft connective tissue diseases. Dordrecht: Springer Netherlands; 2014. pp. 95-105.
37. Micha D, Guo DC, Hilhorst-Hofstee Y, et al. SMAD2 mutations are associated with arterial aneurysms and dissections. Hum Mutat. 2015;36:1145-9.
38. Salzillo C, De Gaspari M, Basso C, Francavilla M, De Leonardis F, Marzullo A. Sudden cardiac death caused by Kawasaki coronary artery vasculitis in a child with Hodgkin’s lymphoma. case report and literature review. Cardiovasc Pathol. 2025;74:107700.
39. Morana E, Guida F, Andreozzi L, et al. Coronary arteries lesions in Kawasaki disease: risk factors in an italian cohort. Biomedicines. 2024;12:2010.
40. Owens AM, Plewa MC. Kawasaki disease. In: StatPearls [Internet]. Treasure Island (FL): StatPearls Publishing; 2024.
42. Khanna S, Garikapati K, Goh DSL, et al. Coronary artery vasculitis: a review of current literature. BMC Cardiovasc Disord. 2021;21:7.
43. Gaspari M, Ascione A, Baldovini C, et al; Italian study group of cardiovascular pathology. Cardiovascular pathology in vasculitis. Pathologica. 2024;116:78-92.
44. González-Gay MÁ, Heras-Recuero E, Blázquez-Sánchez T, et al. Genetics of vasculitis. Best Pract Res Clin Rheumatol. 2024;38:101969.
45. Onouchi Y, Ozaki K, Burns JC, et al. Japan Kawasaki Disease Genome Consortium; US Kawasaki Disease Genetics Consortium. A genome-wide association study identifies three new risk loci for Kawasaki disease. Nat Genet. 2012;44:517-21.
46. Carmona FD, Martín J, González-Gay MA. Genetics of vasculitis. Curr Opin Rheumatol. 2015;27:10-7.
47. Liu J, Yuan P, Pang Y, Su D. ITPKC polymorphism (rs7251246 T > C), coronary artery aneurysms, and thrombosis in patients with Kawasaki disease in a southern han Chinese population. Front Immunol. 2023;14:1184162.
48. Wang Y, Lin K, Zhang L, et al. The rs7404339 AA genotype in CDH5 contributes to increased risks of Kawasaki disease and coronary artery lesions in a southern chinese child population. Front Cardiovasc Med. 2022;9:760982.
50. Fujiwara T, Fujiwara H, Nakano H. Pathological features of coronary arteries in children with Kawasaki disease in which coronary arterial aneurysm was absent at autopsy. Quantitative analysis. Circulation. 1988;78:345-50.
51. Trinidad B, Surmachevska N, Lala V. Takayasu Arteritis. In: StatPearls [Internet]. Treasure Island (FL): StatPearls Publishing; 2024.
52. Somashekar A, Leung YT. Updates in the diagnosis and management of Takayasu’s arteritis. Postgrad Med. 2023;135:14-21.
53. Bhandari S, Butt SRR, Ishfaq A, et al. Pathophysiology, diagnosis, and management of takayasu arteritis: a review of current advances. Cureus. 2023;15:e42667.
54. Misra DP, Singh K, Rathore U, et al. Management of takayasu arteritis. Best Pract Res Clin Rheumatol. 2023;37:101826.
57. Terao C, Yoshifuji H, Kimura A, et al. Two susceptibility loci to takayasu arteritis reveal a synergistic role of the IL12B and HLA-B regions in a Japanese population. Am J Hum Genet. 2013;93:289-97.
58. Renauer PA, Saruhan-Direskeneli G, Coit P, et al. Identification of susceptibility loci in IL6, RPS9/LILRB3, and an intergenic locus on chromosome 21q22 in takayasu arteritis in a genome-wide association study. Arthritis Rheumatol. 2015;67:1361-8.
59. Kauferstein S, Beckmann BM. Postmortem genetic analysis following sudden cardiac death : background, approach, and future. Herzschrittmacherther Elektrophysiol. 2024;35:31-8.
60. Kelly KL, Lin PT, Basso C, et al. Sudden cardiac death in the young: a consensus statement on recommended practices for cardiac examination by pathologists from the society for cardiovascular pathology. Cardiovasc Pathol. 2023;63:107497.
61. Salzillo C, La Verde M, Imparato A, Molitierno R, Lucà S, Pagliuca F, Marzullo A. Cardiovascular diseases in public health: chromosomal abnormalities in congenital heart disease causing sudden cardiac death in children. Medicina. 2024;60:1976.
Cite This Article
How to Cite
Download Citation
Export Citation File:
Type of Import
Tips on Downloading Citation
Citation Manager File Format
Type of Import
Direct Import: When the Direct Import option is selected (the default state), a dialogue box will give you the option to Save or Open the downloaded citation data. Choosing Open will either launch your citation manager or give you a choice of applications with which to use the metadata. The Save option saves the file locally for later use.
Indirect Import: When the Indirect Import option is selected, the metadata is displayed and may be copied and pasted as needed.
About This Article
Copyright
Data & Comments
Data
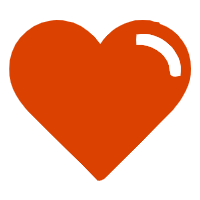
Comments
Comments must be written in English. Spam, offensive content, impersonation, and private information will not be permitted. If any comment is reported and identified as inappropriate content by OAE staff, the comment will be removed without notice. If you have any queries or need any help, please contact us at [email protected].