Population of inflammatory cells in intracranial aneurysm with the special insight to the development of novel diagnostic and therapeutic approaches
Abstract
Intracranial aneurysms (IAs) can cause a lethal subarachnoid hemorrhage after rupture. The prevalence of IA is high in the general public; however, the annual risk for the rupture of an incidentally found lesion is relatively low. Therefore, it is crucial to selectively diagnose rupture-prone IAs among many diagnosed IAs, and properly treat such IAs before rupture. Recent studies using human IA specimens or experimentally-induced IAs in animals have revealed some important findings regarding the role of inflammatory cells infiltrating IA lesions. Currently, IA is considered an inflammatory disease of the intracranial arterial walls. Macrophages are presumably a major type of inflammatory cells regulating the pathogenesis of IAs through the production of a wide range of pro-inflammatory factors. Based on a series of studies, macrophages could be a diagnostic target for rupture-prone IAs. Currently, the potential diagnostic method to detect iron-engulfing macrophages in IA lesions by magnetic resonance imaging is reported. In this review, the authors will summarize the findings regarding the inflammatory cell types present in IA lesions and discuss future prospects for the development of a novel diagnostic method identifying rupture-prone IAs.
Keywords
Introduction
Despite the existence of intensive treatments and modern technical advancements in medical care, subarachnoid hemorrhage caused by the rupture of an intracranial aneurysm (IA) has a poor prognosis with a mortality rate of up to 50%.[1] In addition, subarachnoid hemorrhage can cause sudden death, even in the productive population, making this disease socially important. Given such a devastating outcome and difficulty in treatment once the subarachnoid hemorrhage develops, rupture-preventing treatment of IAs is essential. Currently, many IAs are incidentally found before rupture through a medical checkup of the brain, particularly in developed countries. Indeed, in a Japanese cohort, the majority of unruptured IAs were found incidentally.[2] The detection of unruptured IAs enables prophylactic interventions for the prevention of rupture and the subsequent onset of subarachnoid hemorrhage. Currently, IAs with a high probability of rupture are holistically selected using morphological aspects such as size and shape, anatomical aspects such as location, and other confounding factors which, according to some guidelines and previous cohort studies, increase the likelihood of rupture, such as a previous or family history of subarachnoid hemorrhage, race (Japanese or Finnish), current smoking status, or the presence of hypertension. IAs with a high risk for rupture are surgically treated using microsurgical or endovascular procedures.[3-5] In order to predict the risk of IA rupture more objectively and accurately, a scoring system has been established based on a meta-analysis of 6 prospective cohort studies on the annual rupture risk of IAs.[3,6] However, the lack of a diagnostic method to qualitatively estimate the rupture risk for each IA is currently a major concern in IA treatment. The natural consequence is that IAs with a lower probability of rupture are sometimes surgically treated with a considerable risk for complications, or lesions on the verge of rupture are simply observed, resulting in a devastating outcome. Therefore, a novel qualitative diagnostic method should be established in order to reduce inappropriate decisions regarding surgical intervention.
Another important concern regarding current IA treatment for rupture prevention is the lack of medical treatment (expect for medical care targeting risk factors such as hypertension) for patients with IAs ill-suited for surgery, including patients with small IAs or elderly patients with significant comorbidity.[6] Considering the poor outcome associated with subarachnoid hemorrhage after onset, the intrinsic risk of complications related to surgical manipulations, and the nature of unruptured IAs as asymptomatic lesions, medical IA treatment should be established for patients without surgical indications, or as an alternative to surgical procedures. Currently, statin is considered to be a candidate therapeutic drug for IAs as our previous case-control study demonstrated that statin usage reduced the incidence of subarachnoid hemorrhage due to the rupture of IAs.[7] In addition, a prospective randomized trial examining the inhibitory effect of statins on the progression and rupture of human IAs, known as the Small Unruptured Aneurysm Verification Prevention Effect against Growth of cerebral Aneurysm Study Using Statin study (Japan), is in progress. However, the mechanisms underlying the pathogenesis of IAs need to be further examined in order to develop effective and safe medical treatments. Thus, knowledge regarding the cell types regulating the pathogenesis of IAs is essential to the identification of diagnostic or therapeutic targets. Although the histopathological examination of human IAs demonstrated the presence of hyaline deposits, sub-intimal fibrin deposition, and laminar thrombosis in lesions, particularly in ruptured IAs,[4,8,9] thereby implicating endothelial dysfunction as a potential target for medical therapy, we focus on the inflammatory infiltrates found in IA walls in this short review given previous findings that the inflammatory response is crucial in the pathogenesis of IAs.[10-13]
Inflammatory cells in IA lesions
Histopathological analysis of surgically dissected or autopsy-harvested IA specimens has revealed the presence of inflammatory cells in IA lesions. Kataoka et al.[9] demonstrated an increased presence of inflammatory infiltrating immune cells in ruptured human IAs compared to that in unruptured IAs with a positive correlation between inflammatory infiltrates and degenerative changes in the arterial walls, suggesting a role for inflammatory cells in the rupture of IAs. Inflammatory cells found in human IA lesions include macrophages,[14-16] neutrophils,[17] T lymphocytes[14,15] and mast cells.[16,18,19] Among these types of inflammatory cells, the contribution of macrophages, neutrophils, and mast cells to the pathogenesis of IAs has been supported by experimental studies using animal IA models. Below, we review the evidence for each cell type.
T cells
T cells are a major cell type participating in acquired immunity. T cells are differentiated in the thymus from their precursors mainly into CD4-positive and CD8-positive T cells. These differentiated T cell subsets are then distributed throughout the body and are further differentiated into effective subtypes according to the microenvironment in situ, including CD8-positive T cells as well as T helper 1, 2, and 17 cells (Th1, Th2, and Th17). Some T cells function as a node of acquired immunity and regulate inflammatory responses in a coordinated manner with other cell types in the microenvironment. As expected, T cells play a crucial role in various diseases, including inflammatory and autoimmune diseases.[20] The accumulation of T cells in human IA lesions has been pathologically demonstrated, suggesting the contribution of this cell type to the rupture of IAs.[14,15] Although a recent study has demonstrated the predominance of Th1 and Th17 subsets over Th2 in ruptured IAs,[21] the detailed role of T cells in the pathogenesis of IAs, including which subset/subtype of T cells regulates IA formation and rupture, remains to be elucidated.
Mast cells
Mast cells are characterized by a large number of cytoplasmic granules and are well recognized as mediators of certain types of inflammation including allergic inflammation.[22,23] Mast cells play a role in inflammation through degranulation of cytoplasmic granules, which contain a variety of cytokines and pro-inflammatory factors such as tumor necrosis factor-alpha (TNF-α), interleukin (IL)-1β, IL-3, IL-4, IL-6, IL-8, IL-13, and transforming growth factor-beta (TGF-β).[24-29] Once mast cells are activated, they release large amounts of pro-inflammatory factors from the granules into the extracellular space, resulting in the induction of inflammatory responses within the microenvironment. Mast cells significantly participate in the pathogenesis of various inflammation-related diseases, including vascular diseases such as atherosclerosis,[24,30-33] via the release of pro-inflammatory factors from granules.
In the case of IA, the presence of mast cells in human IA lesions has been demonstrated.[16,19] Hasan et al.[16] further demonstrated that the number of mast cells located in IA lesions is larger for ruptured compared to unruptured IAs, suggesting a role for mast cells in the rupture of IAs. In addition, Ollikainen et al.[19] demonstrated a positive correlation between the number of mast cells present in IA lesions and neovascularization and iron deposition (presumably due to microhemorrhage), suggesting the contribution of mast cells to degenerative changes in the media. However, the exact contribution of mast cells to the pathogenesis of IAs is difficult to discern from studies using human specimens; thus, the pivotal role of mast cells to the pathogenesis of IA has been clarified using animal IA models.[34,35] These studies have demonstrated an increase in the number of mast cells in IA lesions during progression of the disease. In order to demonstrate a causal relationship between the presence of mast cells and IA progression, the researchers treated rats with emedastine difumarate (1-(2-ethoxyethyl)-2-(hexahydro-4-methyl-1H-1,4-diazepin-1-yl)-benzimidazole difumarate) and tranilast (N-(3,4-dimethoxycinnamoyl) anthranilic acid), two widely-used inhibitors for limiting mast cell degranulation. Inhibition of mast cell degranulation effectively reduced lesion size and degenerative changes of the media through the inhibition of inflammatory responses, including reduced NF-κB activation, monocyte chemoattractant protein-1 (MCP-1) expression, macrophage infiltration, matrix metalloproteinases (MMPs) and IL-1β expression, which facilitate pathogenesis.[10,13,36-39] Moreover, in vitro experiments using a primary culture in which vascular smooth muscle cells and mast cells prone to degranulation are co-cultured, confirmed the contribution of mast cell degranulation to the activation of medial smooth muscle cells.[35] Given that factors suppressed by the administration of degranulation inhibitors are known to facilitate IA formation and progression,[10,13,36-39] mast cells or mast cell degranulation inhibitors may be a therapeutic target for IA treatment. It is encouraging that mast cell degranulation-inhibitors are already widely used as anti-allergic drugs; thus, medical therapy targeting mast cells may be possible in near future.
Neutrophils
Neutrophils, defined using surface markers such as CD11b, Ly-6G and Ly-6C, are antigen-presenting cells. This cell type is recruited by chemoattractant factors such as chemokine (C-X-C motif) ligand 1 (CXCL1)[40] and is present in the inflammatory microenvironment, migrating to inflammation sites and exacerbating inflammatory responses by secreting various pro-inflammatory factors in response to cytokines present in situ. Factors released from neutrophils include proteases and digestive enzymes such as myeloperoxidase, collagenase, elastase, and cathepsin.[20] Neutrophil turnover during inflammation is usually rapid; therefore, this cell type is traditionally believed to be a mediator of the acute inflammatory response. However, recent studies have revealed the contribution of neutrophils to the pathogenesis of diseases with long-lasting inflammation, referred to as chronic inflammatory diseases. For example, neutrophils are the most abundant cell type in colitis-associated colon cancer induced in mice and have been shown to be as a major source of cytokines regulating inflammatory responses, including TNF-α and IL-6, which contribute to the transformation/proliferation of cancer cells.[41,42] The crucial contribution of neutrophils to the pathogenesis of cancer has been clarified in a study demonstrating that the genetic depletion of C-X-C chemokine receptor 2, the receptor for CXCL1, resulted in a significant reduction of tumorigenesis.[41]
In case of IAs, the presence of neutrophils in IA lesions has been demonstrated both in human specimens[17] and in animal models.[17,37] In human IAs, myeloperoxidase-positive cells, including neutrophils, are abundantly present in IA lesions.[17] Genetic loss of myeloperoxidase in mice significantly reduces not only the incidence of IAs, but also delays the onset of subarachnoid hemorrhage. This suppressive action due to the loss of myeloperoxidase is accompanied with the suppression of CXCL1 and other pro-inflammatory factors. These results suggest a role for myeloperoxidase, presumably produced by neutrophils, in the incidence and rupture of IAs. Intriguingly, as the loss of myeloperoxidase suppresses CXCL1 expression, myeloperoxidase may contribute to the formation of a positive feedback loop among neutrophils, which amplifies and exacerbates inflammation. Thus, myeloperoxidase may function to form a vicious cycle leading to the progression of IAs. Therefore, myeloperoxidase, or another factor regulating the activity of neutrophils, could be an ideal therapeutic target in the treatment of IAs.
Macrophages
Macrophages, defined by the expression of surface markers including CD11b, CD68, CD163, are also antigen-presenting cells.[43] It is, of course, the major cell type evoking inflammatory responses. The accumulation of macrophages in human IA lesions has been demonstrated. Chyatte et al.[15] demonstrated that number of macrophages was larger in IA lesions than in control arterial walls, and thus proposed a role for macrophages in the formation and rupture of IAs. Frösen et al.[14] analyzed the pathology of surgically-dissected IA walls and found that macrophage infiltration, apoptosis, the loss of endothelial cells, thrombosis in the lumen, and the proliferation of medial smooth muscle cells were more frequently and robustly observed in ruptured IAs than in unruptured IAs, suggesting a role for macrophages in the rupture of IAs. Furthermore, as macrophage infiltration was correlated with the proliferation of medial smooth muscle cells and both were increased in ruptured IAs collected within 12 h after rupture, the authors proposed an additional role of macrophages in the repair of the arterial walls of IA lesions.[14] Hasan et al.[16] examined macrophage subsets (M1 and M2) in IA walls. M1 and M2 are defined according to the expression of several markers with inducible nitric oxide synthase (iNOS) expressed in M1 and CD163 expressed in M2 macrophages.[44] Traditionally, M1 and M2 macrophages are believed to function oppositely in inflammatory settings with M1 exacerbating and M2 ameliorating inflammation. Hasan et al.[16] found that, in unruptured IAs, M1 and M2 populations were similar, but in ruptured IAs, the balance shifted to M1-predominance over M2, indicating a role for M1 in the rupture of IAs. However, careful attention is needed regarding the role of specific macrophage subsets in the rupture of IAs because subarachnoid hemorrhage as the consequence of rupture robustly induces acute inflammation, and thereby presumably shifts the balance among macrophage subsets.
Recent experimental studies using animal IA models have clarified the crucial role of macrophages in IA formation and progression. Using a rat model, Aoki et al.[37] demonstrated that macrophages are the major inflammatory cell type in IA walls with the remaining cell types consisting of T lymphocytes and neutrophils. The authors also demonstrated the presence of mast cells in IA walls in an experimental model; however, macrophages occupied the majority of inflammatory cells.[35] In order to examine the contribution of macrophages to IA formation and progression, mice deficient in monocyte chemoattractant protein-1 (MCP-1), a major chemoattractant for macrophages, were subjected to an IA model.[36] The genetic loss of MCP-1 almost completely inhibited the infiltration of macrophages in lesions and significantly suppressed IA formation to the level seen in un-treated wild type mice.[36] The effect of a genetic loss of MCP-1 on IA formation has also been demonstrated in another study by Kanematsu et al.[45] In addition, Aoki et al.[36] administered the dominant-negative form of MCP-1, known as 7-ND, in a rat IA model by injecting expression plasmid in the femoral muscle, and demonstrate a suppression of IA formation and progression, further confirming the crucial role of MCP-1-mediated macrophage infiltration in IA formation and progression. In the IA walls of MCP-1-deficient mice, the inflammatory response, including NF-κB activation as well as iNOS and MMP-9 induction, was remarkably ameliorated compared with that of wild type mice,[36] supporting the role of macrophages as an inducer of inflammation in lesions. The importance of macrophages in the pathogenesis of IAs is also supported by Kanematsu et al.[45] in which the pharmacological depletion of macrophages using clodronate liposome remarkably suppressed IA formation in mice. MCP-1 expression overlaps with NF-κB in endothelial cells during the early stage of IA formation,[36] indicating that NF-κB-dependent MCP-1 expression and trans-endothelial migration of macrophages occurs via MCP-1. Indeed, mice deficient in the NF-κB p50 subunit show significantly less induction of MCP-1 in lesions, supporting the dependency of NF-κB on MCP-1 expression in lesions.[13] Given that the high wall shear stress loaded on bifurcation sites of intracranial arteries where IA forms is considered to be a trigger of IA formation through extensive studies conducted in mainly 3D-computational fluid dynamics analyses of human IA lesions,[10,46-48] one potential factor triggering NF-κB-dependent MCP-1 expression in endothelial cells is presumably this hemodynamic force. However, further studies are needed in order to corroborate whether macrophages indeed migrate across endothelial cells during IA formation and progression. Given these findings, IA is now considered to be a macrophage-mediated inflammatory disease of the intracranial arteries. Therefore, macrophages could be a target in the development of a novel diagnostic method and in the development of a therapeutic drug.
Macrophage imaging
Given the devastating consequences of an aneurysmal subarachnoid hemorrhage as well as the relatively low annual risk of rupture,[2] it is crucial to appropriately select rupture-prone IAs among many unruptured IAs while avoiding unnecessary surgical intervention through the proper estimation of the rupture risk for each lesion. For example, a qualitative evaluation using non- or minimally-invasive magnetic resonance imaging (MRI) of IA lesions could be a candidate diagnostic method. Given the crucial contribution of macrophage-mediated inflammatory responses to the pathogenesis of IAs,[10,36,45,49] macrophages as well as factors secreted from or factors recruiting macrophages can be considered potential targets for imaging. Macrophages are an antigen-presenting cell; thus, macrophages actively search for and engulf foreign bodies by nature. Through their phagocytic activity, contrast agent-engulfing macrophages can be theoretically visualized using MRI. Indeed, many studies have demonstrated the application of this type of imaging technique for macrophage-mediated pathological conditions or diseases, including infection and atherosclerosis.[50,51] In these studies, ferumoxytol, which is an ultra-small superparamagnetic particle of iron oxide approved by the Food and Drug Administration (FDA) for the treatment of Iron Deficiency Anemia due to chronic renal failure, was applied as a contrast agent. Recently, the use of macrophage imaging for IAs has been reported.[52-55] In a series of reports, Ferumoxytol was intravenously administered to patients with pre-diagnosed unruptured IAs and ferumoxytol-engulfing macrophages were visualized using a T2 star weighted image. In addition, in order to validate the MRI findings, the presence of iron particles was histologically confirmed using Prussian blue staining of CD68+ macrophages in the IA walls identified as macrophage-rich lesions from ferumoxytol-MRI.[52] Intriguingly, anti-inflammatory drugs (non-steroidal anti-inflammatory drugs) decreased signals in this imaging method, which was accompanied with a decrease in the macrophage count within lesions,[53] suggesting that macrophage-imaging using ferumoxytol-MRI can positively reflect macrophage-rich or poor lesions. Importantly, these findings suggest the potential of this imaging method as a surrogate marker to non-invasively monitor disease activity. Unfortunately, ferumoxytol has a risk of a fatal allergic reaction and the FDA has strengthened the warning concerning usage of this drug as a contrast agent for diagnostic purposes. Therefore, a novel contrast agent for macrophage imaging with excellent biocompatibility and adequate safety is needed. In addition, because red blood cells contain a large amount of iron in the hemoglobin, macrophage-imaging using iron-containing particles need to be evaluated after the subtraction of signals derived from red blood cells in the vasculature. Unfortunately, these subtraction procedures are often time-consuming and make interpretation quite difficult. Hence, to overcome the technical limitations related to iron-particle-based macrophage-imaging methods, positive contrast agents such as nano-particles containing gadolinium may be beneficial. Furthermore, with the use of a nano-particle based technique, a novel drug delivery system targeting macrophages may be possible. If so, IAs with abundant macrophage infiltration can be selectively targeted and treated by a cytotoxic agent in order to reduce macrophage-mediated inflammation in IA lesions.
As described above, macrophages have a wide range of subpopulations with different characteristics.[44] In human IA lesions, the M1 and M2 subpopulations are detected using immunostaining.[16] Further, as indicated in the previous report demonstrating a marked predominance of M1 over M2 in ruptured IAs compared to that in unruptured lesions,[16] a particular subpopulation of macrophages may alone contribute to the rupture of IAs. If so, the detection of this specific subpopulation would be helpful in discriminating rupture-prone IAs from stable lesions. Thus, next-generation imaging modalities for subpopulation-specific visualization of macrophages are desired. In addition, MRI contrast agents that target enzymes produced by inflammatory cells may be useful in diagnosing rupture-prone ‘dangerous’ lesions as suggested in a recent review article.[55] For example, myeloperoxidase is an enzyme produced by myeloid-linage cells such as neutrophils. Enzymatic activity of this protein can be monitored by MRI using gadolinium-chelating bis (5-hydroxytrytamide) derivatives of diethylenetetraamine pentaacetic acid as a contrast agent.[56] Hence, if such a kind of contrast agent can be developed for clinical usage, rupture-prone IAs could presumably be differentiated from stable lesions.
Conclusion
The accumulation of histopathological and experimental evidence from human IA specimens and animal IA models has significantly promoted the conceptual understanding of the pathogenesis of IA and has defined IA as a macrophage-mediated chronic inflammatory disease of the arterial walls. This recent advancement in the understanding of IA pathogenesis greatly encourages the development of not only novel diagnostic methods for the detection of rupture-prone IAs, but also the development of medical therapy targeting macrophages. In the near future, the diagnosis and treatment of unruptured IAs will enter a phase of major change. We hope that many patients with this disease will be more properly diagnosed and more safely treated.
Acknowledgments
Authors would like to express our sincere gratitude to all the researchers, collaborators, technical assistants and secretaries contributing to our studies and also to grants supporting our research work.
Financial support and sponsorship
T.A. is supported by the Coordination Fund from JST and Astellas Pharma Inc.
Conflicts of interest
There are no conflicts of interest.
Patient consent
No patient involved.
Ethics approval
This article does not contain any studies with human participants or animals.
REFERENCES
2. Morita A, Kirino T, Hashi K, Aoki N, Fukuhara S, Hashimoto N, Nakayama T, Sakai M, Teramoto A, Tominari S, Yoshimoto T. The natural course of unruptured cerebral aneurysms in a Japanese cohort. N Engl J Med 2012;366:2474-82.
3. Sonobe M, Yamazaki T, Yonekura M, Kikuchi H. Small Unruptured Intracranial Aneurysm Verification Study; SUAVe Study, Japan. Stroke 2010;41:1969-77.
4. Hokari M, Nakayama N, Nishihara H, Houkin K. Pathological findings of saccular cerebral aneurysms-impact of subintimal fibrin deposition on aneurysm rupture. Neurosurg Rev 2015;38:531-40; discussion 40.
5. Etminan N, Brown RJ, Beseoglu K, Juvela S, Raymond J, Morita A, Torner J, Derdeyn C, Raabe A, Mocco J, Korja M, Abdulazim A, Amin-Hanjani S, Al-Shahi Salman R, Barrow D, Bederson J, Bonafe A, Dumont A, Fiorella D, Gruber A, Hankey G, Hasan D, Hoh B, Jabbour P, Kasuya H, Kelly M, Kirkpatrick P, Knuckey N, Koivisto T, Krings T, Lawton M, Marotta T, Mayer S, Mee E, Pereira V, Molyneux A, Morgan M, Mori K, Murayama Y, Nagahiro S, Nakayama N, Niemelä M, Ogilvy C, Pierot L, Rabinstein A, Roos Y, Rinne J, Rosenwasser R, Ronkainen A, Schaller K, Seifert V, Solomon R, Spears J, Steiger H, Vergouwen M, Wanke I, Wermer M, Wong G, Wong J, Zipfel G, Connolly EJ, Steinmetz H, Lanzino G, Pasqualin A, Rüfenacht D, Vajkoczy P, McDougall C, Hänggi D, LeRoux P, Rinkel G, Macdonald R. The unruptured intracranial aneurysm treatment score: a multidisciplinary consensus. Neurology 2015;85:881-9.
6. Greving JP, Wermer MJ, Brown RD Jr. , Morita A, Juvela S, Yonekura M, Ishibashi T, Torner JC, Nakayama T, Rinkel GJ, Algra A. Development of the PHASES score for prediction of risk of rupture of intracranial aneurysms: a pooled analysis of six prospective cohort studies. Lancet Neurol 2014;13:59-66.
7. Yoshimura Y, Murakami Y, Saitoh M, Yokoi T, Aoki T, Miura K, Ueshima H, Nozaki K. Statin use and risk of cerebral aneurysm rupture: a hospital-based case-control study in Japan. J Stroke Cerebrovasc Dis 2014;23:343-8.
8. Tulamo R, Frosen J, Hernesniemi J, Niemela M. Inflammatory changes in the aneurysm wall: a review. J Neurointerv Surg 2010;2:120-30.
9. Kataoka K, Taneda M, Asai T, Kinoshita A, Ito M, Kuroda R. Structural fragility and inflammatory response of ruptured cerebral aneurysms. A comparative study between ruptured and unruptured cerebral aneurysms. Stroke 1999;30:1396-401.
10. Aoki T, Nishimura M. Targeting chronic inflammation in cerebral aneurysms: focusing on NF-kappaB as a putative target of medical therapy. Expert Opin Ther Targets 2010;14:265-73.
11. Fukuda M, Aoki T. Molecular basis for intracranial aneurysm formation. Acta Neurochir Suppl 2015;120:13-5.
12. Aoki T, Narumiya S. Prostaglandins and chronic inflammation. Trends Pharmacol Sci 2012;33:304-11.
13. Aoki T, Kataoka H, Shimamura M, Nakagami H, Wakayama K, Moriwaki T, Ishibashi R, Nozaki K, Morishita R, Hashimoto N. NF-kappaB is a key mediator of cerebral aneurysm formation. Circulation 2007;116:2830-40.
14. Frosen J, Piippo A, Paetau A, Kangasniemi M, Niemela M, Hernesniemi J, Jaaskelainen J. Remodeling of saccular cerebral artery aneurysm wall is associated with rupture: histological analysis of 24 unruptured and 42 ruptured cases. Stroke 2004;35:2287-93.
15. Chyatte D, Bruno G, Desai S, Todor DR. Inflammation and intracranial aneurysms. Neurosurgery 1999;45:1137-46.
16. Hasan D, Chalouhi N, Jabbour P, Hashimoto T. Macrophage imbalance (M1 vs. M2) and upregulation of mast cells in wall of ruptured human cerebral aneurysms: preliminary results. J Neuroinflammation 2012;9:222.
17. Chu Y, Wilson K, Gu H, Wegman-Points L, Dooley SA, Pierce GL, Cheng G, Pena Silva RA, Heistad DD, Hasan D. Myeloperoxidase is increased in human cerebral aneurysms and increases formation and rupture of cerebral aneurysms in mice. Stroke 2015;46:1651-6.
18. Faleiro LC, Machado CR, Gripp A Jr. , Resende RA, Rodrigues PA. Cerebral vasospasm: presence of mast cells in human cerebral arteries after aneurysm rupture. J Neurosurg 1981;54:733-5.
19. Ollikainen E, Tulamo R, Frosen J, Lehti S, Honkanen P, Hernesniemi J, Niemela M, Kovanen PT. Mast cells, neovascularization, and microhemorrhages are associated with saccular intracranial artery aneurysm wall remodeling. J Neuropathol Exp Neurol 2014;73:855-64.
20. Abbas AK, Lichtman AH, Pillai S. Cellular and Molecular Immunology eighth edition. In: editor editors, editor. PA: Elsevier Saunders; 2014.
21. Zhang HF, Zhao MG, Liang GB, Yu CY, He W, Li ZQ, Gao X. Dysregulation of CD4(+) T Cell Subsets in Intracranial Aneurysm. DNA Cell Biol 2016;35:96-103.
22. Krishnaswamy G, Ajitawi O, Chi DS. The human mast cell: an overview. Methods Mol Biol 2006;315:13-34.
23. Stassen M, Hultner L, Muller C, Schmitt E. Mast cells and inflammation. Arch Immunol Ther Exp (Warsz) 2002;50:179-85.
24. Bacci S, Pieri L, Buccoliero AM, Bonelli A, Taddei G, Romagnoli P. Smooth muscle cells, dendritic cells and mast cells are sources of TNFalpha and nitric oxide in human carotid artery atherosclerosis. Thromb Res 2008;122:657-67.
25. Bhattacharyya SP, Drucker I, Reshef T, Kirshenbaum AS, Metcalfe DD, Mekori YA. Activated T lymphocytes induce degranulation and cytokine production by human mast cells following cell-to-cell contact. J Leukoc Biol 1998;63:337-41.
26. Dumitrascu D. Mast cells as potent inflammatory cells. Rom J Intern Med 1996;34:159-72.
27. Kagami S, Kanari H, Suto A, Fujiwara M, Ikeda K, Hirose K, Watanabe N, Iwamoto I, Nakajima H. HMG-CoA reductase inhibitor simvastatin inhibits proinflammatory cytokine production from murine mast cells. Int Arch Allergy Immunol 2008:146 Suppl 1: 61-6.
28. Nakae S, Iikura M, Suto H, Akiba H, Umetsu DT, Dekruyff RH, Saito H, Galli SJ. TIM-1 and TIM-3 enhancement of Th2 cytokine production by mast cells. Blood 2007;110:2565-8.
29. Moller A, Henz BM, Grutzkau A, Lippert U, Aragane Y, Schwarz T, Kruger-Krasagakes S. Comparative cytokine gene expression: regulation and release by human mast cells. Immunology 1998;93:289-95.
30. Ali AS, Lax AS, Liljestrom M, Paakkari I, Ashammakhi N, Kovanen PT, Konttinen YT. Mast cells in atherosclerosis as a source of the cytokine RANKL. Clin Chem Lab Med 2006;44:672-4.
31. Chaldakov GN, Valchanov K, Tonchev A, Ghenev PI. The association of mast cells and atherosclerosis: new insights into mast cells in atherogenesis. Hum Pathol 1995;26:1286.
32. Sun J, Sukhova GK, Wolters PJ, Yang M, Kitamoto S, Libby P, MacFarlane LA, Mallen-St Clair J, Shi GP. Mast cells promote atherosclerosis by releasing proinflammatory cytokines. Nat Med 2007;13:719-24.
33. Sun J, Sukhova GK, Yang M, Wolters PJ, MacFarlane LA, Libby P, Sun C, Zhang Y, Liu J, Ennis TL, Knispel R, Xiong W, Thompson RW, Baxter BT, Shi GP. Mast cells modulate the pathogenesis of elastase-induced abdominal aortic aneurysms in mice. J Clin Invest 2007;117:3359-68.
34. Aoki T, Nishimura M. The development and the use of experimental animal models to study the underlying mechanisms of CA formation. J Biomed Biotechnol 2011;2011:535921.
35. Ishibashi R, Aoki T, Nishimura M, Hashimoto N, Miyamoto S. Contribution of mast cells to cerebral aneurysm formation. Curr Neurovasc Res 2010;7:113-24.
36. Aoki T, Kataoka H, Ishibashi R, Nozaki K, Egashira K, Hashimoto N. Impact of monocyte chemoattractant protein-1 deficiency on cerebral aneurysm formation. Stroke 2009;40:942-51.
37. Aoki T, Kataoka H, Morimoto M, Nozaki K, Hashimoto N. Macrophage-derived matrix metalloproteinase-2 and -9 promote the progression of cerebral aneurysms in rats. Stroke 2007;38:162-9.
38. Moriwaki T, Takagi Y, Sadamasa N, Aoki T, Nozaki K, Hashimoto N. Impaired progression of cerebral aneurysms in interleukin-1beta-deficient mice. Stroke 2006;37:900-5.
39. Kataoka H, Aoki T. Molecular basis for the development of intracranial aneurysm. Expert Rev Neurother 2010;10:173-87.
40. Oppenheim JJ, Zachariae CO, Mukaida N, Matsushima K. Properties of the novel proinflammatory supergene "intercrine" cytokine family. Annu Rev Immunol 1991;9:617-48.
41. Katoh H, Wang D, Daikoku T, Sun H, Dey SK, Dubois RN. CXCR2-expressing myeloid-derived suppressor cells are essential to promote colitis-associated tumorigenesis. Cancer Cell 2013;24:631-44.
42. Ma X, Aoki T, Tsuruyama T, Narumiya S. Definition of Prostaglandin E2-EP2 Signals in the Colon Tumor Microenvironment That Amplify Inflammation and Tumor Growth. Cancer Res 2015;75:2822-32.
43. Murray PJ, Wynn TA. Protective and pathogenic functions of macrophage subsets. Nat Rev Immunol 2011;11:723-37.
44. Murray PJ, Allen JE, Biswas SK, Fisher EA, Gilroy DW, Goerdt S, Gordon S, Hamilton JA, Ivashkiv LB, Lawrence T, Locati M, Mantovani A, Martinez FO, Mege JL, Mosser DM, Natoli G, Saeij JP, Schultze JL, Shirey KA, Sica A, Suttles J, Udalova I, van Ginderachter JA, Vogel SN, Wynn TA. Macrophage activation and polarization: nomenclature and experimental guidelines. Immunity 2014;41:14-20.
45. Kanematsu Y, Kanematsu M, Kurihara C, Tada Y, Tsou TL, van Rooijen N, Lawton MT, Young WL, Liang EI, Nuki Y, Hashimoto T. Critical roles of macrophages in the formation of intracranial aneurysm. Stroke 2011;42:173-8.
46. Shojima M, Oshima M, Takagi K, Torii R, Hayakawa M, Katada K, Morita A, Kirino T. Magnitude and role of wall shear stress on cerebral aneurysm: computational fluid dynamic study of 20 middle cerebral artery aneurysms. Stroke 2004;35:2500-5.
47. Alfano JM, Kolega J, Natarajan SK, Xiang J, Paluch RA, Levy EI, Siddiqui AH, Meng H. Intracranial aneurysms occur more frequently at bifurcation sites that typically experience higher hemodynamic stresses. Neurosurgery 2013;73:497-505.
48. Dolan JM, Kolega J, Meng H. High wall shear stress and spatial gradients in vascular pathology: a review. Ann Biomed Eng 2013;41:1411-27.
49. Aoki T, Narumiya S. Prostaglandins and chronic inflammation. Trends Pharmacol Sci 2012;33:304-11.
50. Neuwelt A, Sidhu N, Hu CA, Mlady G, Eberhardt SC, Sillerud LO. Iron-based superparamagnetic nanoparticle contrast agents for MRI of infection and inflammation. AJR Am J Roentgenol 2015;204:W302-13.
51. Zhao X, Zhao H, Chen Z, Lan M. Ultrasmall superparamagnetic iron oxide nanoparticles for magnetic resonance imaging contrast agent. J Nanosci Nanotechnol 2014;14:210-20.
52. Hasan DM, Mahaney KB, Magnotta VA, Kung DK, Lawton MT, Hashimoto T, Winn HR, Saloner D, Martin A, Gahramanov S, Dosa E, Neuwelt E, Young WL. Macrophage imaging within human cerebral aneurysms wall using ferumoxytol-enhanced MRI: a pilot study. Arterioscler Thromb Vasc Biol 2012;32:1032-8.
53. Hasan DM, Chalouhi N, Jabbour P, Dumont AS, Kung DK, Magnotta VA, Young WL, Hashimoto T, Richard Winn H, Heistad D. Evidence that acetylsalicylic acid attenuates inflammation in the walls of human cerebral aneurysms: preliminary results. J Am Heart Assoc 2013;2:e000019.
54. Hasan D, Chalouhi N, Jabbour P, Dumont AS, Kung DK, Magnotta VA, Young WL, Hashimoto T, Winn HR, Heistad D. Early change in ferumoxytol-enhanced magnetic resonance imaging signal suggests unstable human cerebral aneurysm: a pilot study. Stroke 2012;43:3258-65.
55. Chalouhi N, Jabbour P, Magnotta V, Hasan D. Molecular imaging of cerebrovascular lesions. Transl Stroke Res 2014;5:260-8.
Cite This Article
How to Cite
Koseki, H.; Aoki T. Population of inflammatory cells in intracranial aneurysm with the special insight to the development of novel diagnostic and therapeutic approaches. Neurosciences. 2016, 3, 173-9. http://dx.doi.org/10.20517/2347-8659.2016.05
Download Citation
Export Citation File:
Type of Import
Tips on Downloading Citation
Citation Manager File Format
Type of Import
Direct Import: When the Direct Import option is selected (the default state), a dialogue box will give you the option to Save or Open the downloaded citation data. Choosing Open will either launch your citation manager or give you a choice of applications with which to use the metadata. The Save option saves the file locally for later use.
Indirect Import: When the Indirect Import option is selected, the metadata is displayed and may be copied and pasted as needed.
About This Article
Copyright
Author Biographies
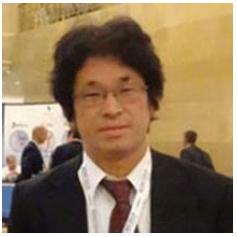
Data & Comments
Data
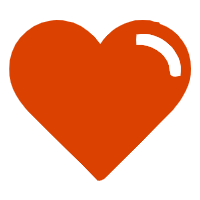
Comments
Comments must be written in English. Spam, offensive content, impersonation, and private information will not be permitted. If any comment is reported and identified as inappropriate content by OAE staff, the comment will be removed without notice. If you have any queries or need any help, please contact us at support@oaepublish.com.